Insights from the sea: structural biology of marine polyketide synthases
- PMID:22498975
- PMCID: PMC3709256
- DOI: 10.1039/c2np20016c
Insights from the sea: structural biology of marine polyketide synthases
Abstract
The world's oceans are a rich source of natural products with extremely interesting chemistry. Biosynthetic pathways have been worked out for a few, and the story is being enriched with crystal structures of interesting pathway enzymes. By far, the greatest number of structural insights from marine biosynthetic pathways has originated with studies of curacin A, a poster child for interesting marine chemistry with its cyclopropane and thiazoline rings, internal cis double bond, and terminal alkene. Using the curacin A pathway as a model, structural details are now available for a novel loading enzyme with remarkable dual decarboxylase and acetyltransferase activities, an Fe(2+)/α-ketoglutarate-dependent halogenase that dictates substrate binding order through conformational changes, a decarboxylase that establishes regiochemistry for cyclopropane formation, and a thioesterase with specificity for β-sulfated substrates that lead to terminal alkene offloading. The four curacin A pathway dehydratases reveal an intrinsic flexibility that may accommodate bulky or stiff polyketide intermediates. In the salinosporamide A pathway, active site volume determines the halide specificity of a halogenase that catalyzes for the synthesis of a halogenated building block. Structures of a number of putative polyketide cyclases may help in understanding reaction mechanisms and substrate specificities although their substrates are presently unknown.
Figures
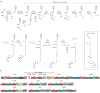
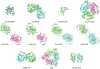
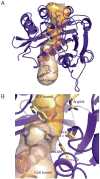
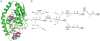
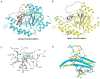
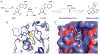
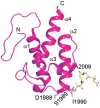
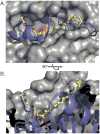
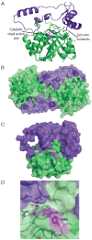
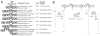
Similar articles
- Terminal alkene formation by the thioesterase of curacin A biosynthesis: structure of a decarboxylating thioesterase.Gehret JJ, Gu L, Gerwick WH, Wipf P, Sherman DH, Smith JL.Gehret JJ, et al.J Biol Chem. 2011 Apr 22;286(16):14445-54. doi: 10.1074/jbc.M110.214635. Epub 2011 Feb 27.J Biol Chem. 2011.PMID:21357626Free PMC article.
- Crystal structures of dehydratase domains from the curacin polyketide biosynthetic pathway.Akey DL, Razelun JR, Tehranisa J, Sherman DH, Gerwick WH, Smith JL.Akey DL, et al.Structure. 2010 Jan 13;18(1):94-105. doi: 10.1016/j.str.2009.10.018.Structure. 2010.PMID:20152156Free PMC article.
- Anatomy of the β-branching enzyme of polyketide biosynthesis and its interaction with an acyl-ACP substrate.Maloney FP, Gerwick L, Gerwick WH, Sherman DH, Smith JL.Maloney FP, et al.Proc Natl Acad Sci U S A. 2016 Sep 13;113(37):10316-21. doi: 10.1073/pnas.1607210113. Epub 2016 Aug 29.Proc Natl Acad Sci U S A. 2016.PMID:27573844Free PMC article.
- Structural diversity and chemical synthesis of peroxide and peroxide-derived polyketide metabolites from marine sponges.Norris MD, Perkins MV.Norris MD, et al.Nat Prod Rep. 2016 Jul 28;33(7):861-80. doi: 10.1039/c5np00142k. Epub 2016 May 10.Nat Prod Rep. 2016.PMID:27163115Review.
- The Structural Enzymology of Iterative Aromatic Polyketide Synthases: A Critical Comparison with Fatty Acid Synthases.Tsai SC.Tsai SC.Annu Rev Biochem. 2018 Jun 20;87:503-531. doi: 10.1146/annurev-biochem-063011-164509.Annu Rev Biochem. 2018.PMID:29925265Review.
Cited by
- Recent advances in the discovery and combinatorial biosynthesis of microbial 14-membered macrolides and macrolactones.Park JW, Yoon YJ.Park JW, et al.J Ind Microbiol Biotechnol. 2019 Mar;46(3-4):445-458. doi: 10.1007/s10295-018-2095-4. Epub 2018 Nov 10.J Ind Microbiol Biotechnol. 2019.PMID:30415291Review.
- Protein-protein interactions in "cis-AT" polyketide synthases.Dodge GJ, Maloney FP, Smith JL.Dodge GJ, et al.Nat Prod Rep. 2018 Oct 17;35(10):1082-1096. doi: 10.1039/c8np00058a.Nat Prod Rep. 2018.PMID:30188553Free PMC article.Review.
- Description of Agathobaculum massiliense sp. nov., a new bacterial species prevalent in the human gut and predicted to produce indole and tryptophan based on genomic analysis.Magdy Wasfy R, Zoaiter M, Bilen M, Tidjani Alou M, Lo CI, Bellali S, Caputo A, Alibar S, Andrieu C, Raoult D, Fournier PE, Million M.Magdy Wasfy R, et al.Antonie Van Leeuwenhoek. 2023 Jun;116(6):541-555. doi: 10.1007/s10482-023-01824-1. Epub 2023 Apr 8.Antonie Van Leeuwenhoek. 2023.PMID:37029880
- PKS-NRPS Enzymology and Structural Biology: Considerations in Protein Production.Skiba MA, Maloney FP, Dan Q, Fraley AE, Aldrich CC, Smith JL, Brown WC.Skiba MA, et al.Methods Enzymol. 2018;604:45-88. doi: 10.1016/bs.mie.2018.01.035. Epub 2018 Mar 16.Methods Enzymol. 2018.PMID:29779664Free PMC article.
- Probing the structure and function of acyl carrier proteins to unlock the strategic redesign of type II polyketide biosynthetic pathways.Sulpizio A, Crawford CEW, Koweek RS, Charkoudian LK.Sulpizio A, et al.J Biol Chem. 2021 Jan-Jun;296:100328. doi: 10.1016/j.jbc.2021.100328. Epub 2021 Jan 23.J Biol Chem. 2021.PMID:33493513Free PMC article.Review.
References
Publication types
MeSH terms
Substances
Related information
Grants and funding
LinkOut - more resources
Full Text Sources