Clinical parameters affect the structure and function of superficial pyramidal neurons in the adult human neocortex
- PMID:39474044
- PMCID: PMC11518857
- DOI: 10.1093/braincomms/fcae351
Clinical parameters affect the structure and function of superficial pyramidal neurons in the adult human neocortex
Abstract
The interplay between neuronal structure and function underpins the dynamic nature of neocortical networks. Despite extensive studies in animal models, our understanding of structure-function interrelations in the adult human brain remains incomplete. Recent methodological advances have facilitated the functional analysis of individual neurons within the human neocortex, providing a new understanding of fundamental brain processes. However, the factors contributing to patient-specific neuronal properties have not been thoroughly explored. In this observational study, we investigated the structural and functional variability of superficial pyramidal neurons in the adult human neocortex. Using whole-cell patch-clamp recordings andpost hoc analyses of dendritic spine morphology in acute neocortical slice preparations from surgical resections of seven patients, we assessed age-related effects on excitatory neurotransmission, membrane properties and dendritic spine morphologies. These results specify age as an endogenous factor that might affect the structural and functional properties of superficial pyramidal neurons.
Keywords: excitatory synapses; human dendritic spines; human neocortex.
© The Author(s) 2024. Published by Oxford University Press on behalf of the Guarantors of Brain.
Conflict of interest statement
The authors report no competing interests.
Figures
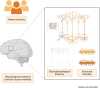
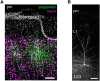
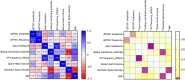
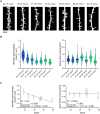
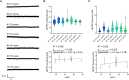
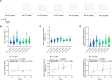
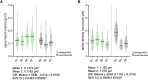
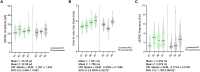
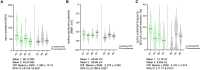
Similar articles
- All-trans retinoic acid induces synaptic plasticity in human cortical neurons.Lenz M, Kruse P, Eichler A, Straehle J, Beck J, Deller T, Vlachos A.Lenz M, et al.Elife. 2021 Mar 30;10:e63026. doi: 10.7554/eLife.63026.Elife. 2021.PMID:33781382Free PMC article.
- In vivo two-photon uncaging of glutamate revealing the structure-function relationships of dendritic spines in the neocortex of adult mice.Noguchi J, Nagaoka A, Watanabe S, Ellis-Davies GC, Kitamura K, Kano M, Matsuzaki M, Kasai H.Noguchi J, et al.J Physiol. 2011 May 15;589(Pt 10):2447-57. doi: 10.1113/jphysiol.2011.207100. Epub 2011 Mar 28.J Physiol. 2011.PMID:21486811Free PMC article.
- Distribution and function of HCN channels in the apical dendritic tuft of neocortical pyramidal neurons.Harnett MT, Magee JC, Williams SR.Harnett MT, et al.J Neurosci. 2015 Jan 21;35(3):1024-37. doi: 10.1523/JNEUROSCI.2813-14.2015.J Neurosci. 2015.PMID:25609619Free PMC article.
- The neocortex. An overview of its evolutionary development, structural organization and synaptology.Nieuwenhuys R.Nieuwenhuys R.Anat Embryol (Berl). 1994 Oct;190(4):307-37. doi: 10.1007/BF00187291.Anat Embryol (Berl). 1994.PMID:7840420Review.
- Anatomical and functional differentiation of glutamatergic synaptic innervation in the neocortex.Wang Y, Gupta A, Markram H.Wang Y, et al.J Physiol Paris. 1999 Sep-Oct;93(4):305-17. doi: 10.1016/s0928-4257(00)80059-5.J Physiol Paris. 1999.PMID:10574120Review.
References
Related information
LinkOut - more resources
Full Text Sources