Role of volcanism and impact heating in mass extinction climate shifts
- PMID:38688982
- PMCID: PMC11061309
- DOI: 10.1038/s41598-024-60467-y
Role of volcanism and impact heating in mass extinction climate shifts
Abstract
This study investigates the mechanisms underlying the varied climate changes witnessed during mass extinctions in the Phanerozoic Eon. Climate shifts during mass extinctions have manifested as either predominant global cooling or predominant warming, yet the causes behind these occurrences remain unclear. We emphasize the significance of sedimentary rock temperature in comprehending these climate shifts. Our research reveals that low-temperature heating of sulfide leads to global cooling through the release of sulfur dioxide (SO2), while intermediate-temperature heating of hydrocarbons and carbonates releases substantial carbon dioxide (CO2), contributing to global warming. High-temperature heating additionally generates SO2 from sulfate, further contributing to global cooling. Different degrees of contact heating of the host rock can lead to different dominant volatile gas emissions, crucially driving either warming or cooling. Moreover, medium to high-temperature shock-heating resulting from asteroid impacts produces soot from hydrocarbons, also contributing to global cooling. Large-scale volcanic activity and asteroid impacts are both events that heat rocks, emitting the same gases and particles, causing climate changes. The findings elucidate the critical role of heating temperature and heating time in understanding major climate changes during mass extinctions.
Keywords: Asteroid impact; Climate variations; Emitted gases; Heating temperature; Mass extinction; Volcanic activity.
© 2024. The Author(s).
Conflict of interest statement
The author declares no competing interests.
Figures
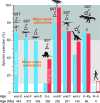
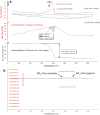
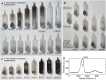
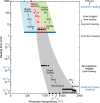
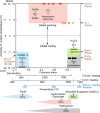
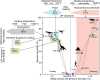
Similar articles
- Asteroid impact, not volcanism, caused the end-Cretaceous dinosaur extinction.Chiarenza AA, Farnsworth A, Mannion PD, Lunt DJ, Valdes PJ, Morgan JV, Allison PA.Chiarenza AA, et al.Proc Natl Acad Sci U S A. 2020 Jul 21;117(29):17084-17093. doi: 10.1073/pnas.2006087117. Epub 2020 Jun 29.Proc Natl Acad Sci U S A. 2020.PMID:32601204Free PMC article.
- Energy, volatile production, and climatic effects of the Chicxulub Cretaceous/Tertiary impact.Pope KO, Baines KH, Ocampo AC, Ivanov BA.Pope KO, et al.J Geophys Res. 1997 Sep 25;102(E9):21645-64. doi: 10.1029/97je01743.J Geophys Res. 1997.PMID:11541145
- Theory and classification of mass extinction causation.Algeo TJ, Shen J.Algeo TJ, et al.Natl Sci Rev. 2023 Sep 8;11(1):nwad237. doi: 10.1093/nsr/nwad237. eCollection 2024 Jan.Natl Sci Rev. 2023.PMID:38116094Free PMC article.Review.
- Thresholds of temperature change for mass extinctions.Song H, Kemp DB, Tian L, Chu D, Song H, Dai X.Song H, et al.Nat Commun. 2021 Aug 4;12(1):4694. doi: 10.1038/s41467-021-25019-2.Nat Commun. 2021.PMID:34349121Free PMC article.
- A Review of Studies Involving the Effects of Climate Change on the Energy Consumption for Building Heating and Cooling.Li Y, Wang W, Wang Y, Xin Y, He T, Zhao G.Li Y, et al.Int J Environ Res Public Health. 2020 Dec 23;18(1):40. doi: 10.3390/ijerph18010040.Int J Environ Res Public Health. 2020.PMID:33374644Free PMC article.Review.
References
- Svensen H, et al. Siberian gas venting and the end-Permian environmental crisis. Earth Planet. Sci. Lett. 2009;277:490–500. doi: 10.1016/j.epsl.2008.11.015. - DOI
- Bond DPG, Grasby SE. On the causes of mass extinctions. Palaeogeogr. Plaeoclimatol. Palaeoecol. 2017;478:3–29. doi: 10.1016/j.palaeo.2016.11.005. - DOI
- Rampino MR, Caldeira K, Prokoph A. What causes mass extinctions? Large asteroid/comet impacts, flood-basalt volcanism, and ocean anoxia—Correlations and cycles. Geol. Soc. Am. Spec. Pap. 2019;542:271–302.
- Kaiho K. Relationship between extinction magnitude and climate change during major marine and terrestrial animal crises. Biogeosciences. 2022;19:3369–3380. doi: 10.5194/bg-19-3369-2022. - DOI
Related information
Grants and funding
LinkOut - more resources
Full Text Sources