Theory and classification of mass extinction causation
- PMID:38116094
- PMCID: PMC10727847
- DOI: 10.1093/nsr/nwad237
Theory and classification of mass extinction causation
Abstract
Theory regarding the causation of mass extinctions is in need of systematization, which is the focus of this contribution. Every mass extinction has both an ultimate cause, i.e. the trigger that leads to various climato-environmental changes, and one or more proximate cause(s), i.e. the specific climato-environmental changes that result in elevated biotic mortality. With regard to ultimate causes, strong cases can be made that bolide (i.e. meteor) impacts, large igneous province (LIP) eruptions and bioevolutionary events have each triggered one or more of the Phanerozoic Big Five mass extinctions, and that tectono-oceanic changes have triggered some second-order extinction events. Apart from bolide impacts, other astronomical triggers (e.g. solar flares, gamma bursts and supernova explosions) remain entirely in the realm of speculation. With regard to proximate mechanisms, most extinctions are related to either carbon-release or carbon-burial processes, the former being associated with climatic warming, ocean acidification, reduced marine productivity and lower carbonate δ13C values, and the latter with climatic cooling, increased marine productivity and higher carbonate δ13C values. Environmental parameters such as marine redox conditions and terrestrial weathering intensity do not show consistent relationships with carbon-cycle changes. In this context, mass extinction causation can be usefully classified using a matrix of ultimate and proximate factors. Among the Big Five mass extinctions, the end-Cretaceous biocrisis is an example of a bolide-triggered carbon-release event, the end-Permian and end-Triassic biocrises are examples of LIP-triggered carbon-release events, and the Late Ordovician and Late Devonian biocrises are examples of bioevolution-triggered carbon-burial events. Whereas the bolide-impact and LIP-eruption mechanisms appear to invariably cause carbon release, bioevolutionary triggers can result in variable carbon-cycle changes, e.g. carbon burial during the Late Ordovician and Late Devonian events, carbon release associated with modern anthropogenic climate warming, and little to no carbon-cycle impact due to certain types of ecosystem change (e.g. the advent of the first predators around the end-Ediacaran; the appearance of Paleolithic human hunters in Australasia and the Americas). Broadly speaking, studies of mass extinction causation have suffered from insufficiently critical thinking-an impartial survey of the extant evidence shows that (i) hypotheses of a common ultimate cause (e.g. bolide impacts or LIP eruptions) for all Big Five mass extinctions are suspect given manifest differences in patterns of environmental and biotic change among them; (ii) the Late Ordovician and Late Devonian events were associated with carbon burial and long-term climatic cooling, i.e. changes that are inconsistent with a bolide-impact or LIP-eruption mechanism; and (iii) claims of periodicity in Phanerozoic mass extinctions depended critically on the now-disproven idea that they shared a common extrinsic trigger (i.e. bolide impacts).
Keywords: biocrisis; bioevolutionary event; bolide; large igneous province; proximate cause; ultimate cause.
© The Author(s) 2023. Published by Oxford University Press on behalf of China Science Publishing & Media Ltd.
Figures
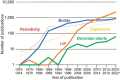
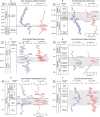
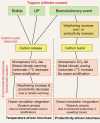
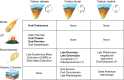
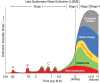
Similar articles
- Biotic and environmental dynamics through the Late Jurassic-Early Cretaceous transition: evidence for protracted faunal and ecological turnover.Tennant JP, Mannion PD, Upchurch P, Sutton MD, Price GD.Tennant JP, et al.Biol Rev Camb Philos Soc. 2017 May;92(2):776-814. doi: 10.1111/brv.12255. Epub 2016 Feb 17.Biol Rev Camb Philos Soc. 2017.PMID:26888552Free PMC article.
- Volcanic related methylmercury poisoning as the possible driver of the end-Devonian Mass Extinction.Rakociński M, Marynowski L, Pisarzowska A, Bełdowski J, Siedlewicz G, Zatoń M, Perri MC, Spalletta C, Schönlaub HP.Rakociński M, et al.Sci Rep. 2020 Apr 30;10(1):7344. doi: 10.1038/s41598-020-64104-2.Sci Rep. 2020.PMID:32355245Free PMC article.
- Biogeochemical significance of pelagic ecosystem function: an end-Cretaceous case study.Henehan MJ, Hull PM, Penman DE, Rae JW, Schmidt DN.Henehan MJ, et al.Philos Trans R Soc Lond B Biol Sci. 2016 May 19;371(1694):20150510. doi: 10.1098/rstb.2015.0510.Philos Trans R Soc Lond B Biol Sci. 2016.PMID:27114586Free PMC article.
- Eutrophication, microbial-sulfate reduction and mass extinctions.Schobben M, Stebbins A, Ghaderi A, Strauss H, Korn D, Korte C.Schobben M, et al.Commun Integr Biol. 2015 Dec 4;9(1):e1115162. doi: 10.1080/19420889.2015.1115162. eCollection 2016 Jan-Feb.Commun Integr Biol. 2015.PMID:27066181Free PMC article.Review.
- The Minderoo-Monaco Commission on Plastics and Human Health.Landrigan PJ, Raps H, Cropper M, Bald C, Brunner M, Canonizado EM, Charles D, Chiles TC, Donohue MJ, Enck J, Fenichel P, Fleming LE, Ferrier-Pages C, Fordham R, Gozt A, Griffin C, Hahn ME, Haryanto B, Hixson R, Ianelli H, James BD, Kumar P, Laborde A, Law KL, Martin K, Mu J, Mulders Y, Mustapha A, Niu J, Pahl S, Park Y, Pedrotti ML, Pitt JA, Ruchirawat M, Seewoo BJ, Spring M, Stegeman JJ, Suk W, Symeonides C, Takada H, Thompson RC, Vicini A, Wang Z, Whitman E, Wirth D, Wolff M, Yousuf AK, Dunlop S.Landrigan PJ, et al.Ann Glob Health. 2023 Mar 21;89(1):23. doi: 10.5334/aogh.4056. eCollection 2023.Ann Glob Health. 2023.PMID:36969097Free PMC article.Review.
Cited by
- Deep-time mass extinction helps understand the current biotic crisis.Xie S.Xie S.Natl Sci Rev. 2023 Dec 20;11(1):nwad322. doi: 10.1093/nsr/nwad322. eCollection 2024 Jan.Natl Sci Rev. 2023.PMID:38250025Free PMC article.No abstract available.
References
- Rudwick MJ. Georges Cuvier, Fossil Bones, and Geological Catastrophes: New Translations and Interpretations of the Primary Texts. Chicago: University of Chicago Press, 2008.
- Algeo TJ, Berner RA, Maynard JBet al. . Late Devonian oceanic anoxic events and biotic crises: “rooted” in the evolution of vascular land plants? GSA Today 1995; 5: 45–66.
- Bambach RK. Phanerozoic biodiversity mass extinctions. Annu Rev Earth Planet Sci 2006; 34: 127–55.10.1146/annurev.earth.33.092203.122654 - DOI
Publication types
Related information
LinkOut - more resources
Full Text Sources