Autonomous DNA molecular motor tailor-designed to navigate DNA origami surface for fast complex motion and advanced nanorobotics
- PMID:37738343
- PMCID: PMC10516491
- DOI: 10.1126/sciadv.adi8444
Autonomous DNA molecular motor tailor-designed to navigate DNA origami surface for fast complex motion and advanced nanorobotics
Abstract
Nanorobots powered by designed DNA molecular motors on DNA origami platforms are vigorously pursued but still short of fully autonomous and sustainable operation, as the reported systems rely on manually operated or autonomous but bridge-burning molecular motors. Expanding DNA nanorobotics requires origami-based autonomous non-bridge-burning motors, but such advanced artificial molecular motors are rare, and their integration with DNA origami remains a challenge. Here, we report an autonomous non-bridge-burning DNA motor tailor-designed for a triangle DNA origami substrate. This is a translational bipedal molecular motor but demonstrates effective translocation on both straight and curved segments of a self-closed circular track on the origami, including sharp ~90° turns by a single hand-over-hand step. The motor is highly directional and attains a record-high speed among the autonomous artificial molecular motors reported to date. The resultant DNA motor-origami system, with its complex translational-rotational motion and big nanorobotic capacity, potentially offers a self-contained "seed" nanorobotic platform to automate or scale up many applications.
Figures
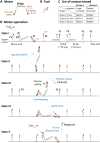
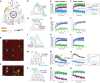
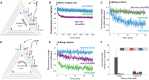
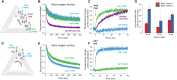
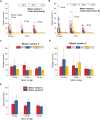
Similar articles
- A light-operated integrated DNA walker-origami system beyond bridge burning.Liu XR, Loh IY, Siti W, Too HL, Anderson T, Wang Z.Liu XR, et al.Nanoscale Horiz. 2023 May 30;8(6):827-841. doi: 10.1039/d2nh00565d.Nanoscale Horiz. 2023.PMID:37038716
- Single-molecule mechanical study of an autonomous artificial translational molecular motor beyond bridge-burning design.Hu X , Zhao X , Loh IY , Yan J , Wang Z .Hu X , et al.Nanoscale. 2021 Aug 21;13(31):13195-13207. doi: 10.1039/d1nr02296b. Epub 2021 Jul 27.Nanoscale. 2021.PMID:34477726
- Track-walking molecular motors: a new generation beyond bridge-burning designs.Wang Z , Hou R , Loh IY .Wang Z , et al.Nanoscale. 2019 May 16;11(19):9240-9263. doi: 10.1039/c9nr00033j.Nanoscale. 2019.PMID:31062798Review.
- A bipedal DNA motor that travels back and forth between two DNA origami tiles.Liber M, Tomov TE, Tsukanov R, Berger Y, Nir E.Liber M, et al.Small. 2015 Feb 4;11(5):568-75. doi: 10.1002/smll.201402028. Epub 2014 Sep 18.Small. 2015.PMID:25236793
- Engineering defined motor ensembles with DNA origami.Goodman BS, Reck-Peterson SL.Goodman BS, et al.Methods Enzymol. 2014;540:169-88. doi: 10.1016/B978-0-12-397924-7.00010-8.Methods Enzymol. 2014.PMID:24630107Review.
References
- N. C. Seeman, Nanotechnology and the double helix. Sci. Am. 290, 64–75 (2004). - PubMed
- Z. Wang, R. Hou, I. Y. Loh, Track-walking molecular motors: A new generation beyond bridge-burning designs. Nanoscale 11, 9240–9263 (2019). - PubMed
- P. W. K. Rothemund, Folding DNA to create nanoscale shapes and patterns. Nature 440, 297–302 (2006). - PubMed
- S. F. J. Wickham, M. Endo, Y. Katsuda, K. Hidaka, J. Bath, H. Sugiyama, A. J. Turberfield, Direct observation of stepwise movement of a synthetic molecular transporter. Nat. Nanotechnol. 6, 166–169 (2011). - PubMed
MeSH terms
Substances
Related information
LinkOut - more resources
Full Text Sources