Genetically encoded tools for in vivo G-protein-coupled receptor agonist detection at cellular resolution
- PMID:36446954
- PMCID: PMC9708909
- DOI: 10.1002/ctm2.1124
Genetically encoded tools for in vivo G-protein-coupled receptor agonist detection at cellular resolution
Abstract
G-protein-coupled receptors (GPCRs) are the most abundant receptor type in the human body and are responsible for regulating many physiological processes, such as sensation, cognition, muscle contraction and metabolism. Further, GPCRs are widely expressed in the brain where their agonists make up a large number of neurotransmitters and neuromodulators. Due to the importance of GPCRs in human physiology, genetically encoded sensors have been engineered to detect GPCR agonists at cellular resolution in vivo. These sensors can be placed into two main categories: those that offer real-time information on the signalling dynamics of GPCR agonists and those that integrate the GPCR agonist signal into a permanent, quantifiable mark that can be used to detect GPCR agonist localisation in a large brain area. In this review, we discuss the various designs of real-time and integration sensors, their advantages and limitations, and some in vivo applications. We also discuss the potential of using real-time and integrator sensors together to identify neuronal circuits affected by endogenous GPCR agonists and perform detailed characterisations of the spatiotemporal dynamics of GPCR agonist release in those circuits. By using these sensors together, the overall knowledge of GPCR-mediated signalling can be expanded.
Keywords: GPCR; genetically encoded sensor; neuromodulation; neurotransmission.
© 2022 The Authors. Clinical and Translational Medicine published by John Wiley & Sons Australia, Ltd on behalf of Shanghai Institute of Clinical Bioinformatics.
Conflict of interest statement
The authors declare they have no conflicts of interest.
Figures
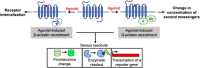
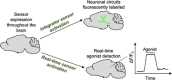
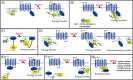

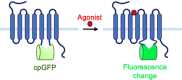
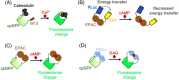
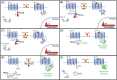
Similar articles
- Single-chain fluorescent integrators for mapping G-protein-coupled receptor agonists.Kroning K, Gannot N, Li X, Putansu A, Zhou G, Sescil J, Shen J, Wilson A, Fiel H, Li P, Wang W.Kroning K, et al.Proc Natl Acad Sci U S A. 2024 Apr 30;121(18):e2307090121. doi: 10.1073/pnas.2307090121. Epub 2024 Apr 22.Proc Natl Acad Sci U S A. 2024.PMID:38648487Free PMC article.
- G-protein-coupled receptor-based sensors for imaging neurochemicals with high sensitivity and specificity.Jing M, Zhang Y, Wang H, Li Y.Jing M, et al.J Neurochem. 2019 Nov;151(3):279-288. doi: 10.1111/jnc.14855. Epub 2019 Oct 13.J Neurochem. 2019.PMID:31419844Free PMC article.Review.
- Single-chain fluorescent integrators for mapping G-protein-coupled receptor agonists.Kroning K, Gannot N, Li X, Zhou G, Sescil J, Putansu A, Shen J, Wilson A, Fiel H, Li P, Wang W.Kroning K, et al.bioRxiv [Preprint]. 2023 Jun 4:2023.05.31.543062. doi: 10.1101/2023.05.31.543062.bioRxiv. 2023.Update in:Proc Natl Acad Sci U S A. 2024 Apr 30;121(18):e2307090121. doi: 10.1073/pnas.2307090121.PMID:37398137Free PMC article.Updated.Preprint.
- Genetically encoded intrabody sensors report the interaction and trafficking of β-arrestin 1 upon activation of G-protein-coupled receptors.Baidya M, Kumari P, Dwivedi-Agnihotri H, Pandey S, Sokrat B, Sposini S, Chaturvedi M, Srivastava A, Roy D, Hanyaloglu AC, Bouvier M, Shukla AK.Baidya M, et al.J Biol Chem. 2020 Jul 24;295(30):10153-10167. doi: 10.1074/jbc.RA120.013470. Epub 2020 May 21.J Biol Chem. 2020.PMID:32439801Free PMC article.
- Illuminating the brain-genetically encoded single wavelength fluorescent biosensors to unravel neurotransmitter dynamics.Kubitschke M, Masseck OA.Kubitschke M, et al.Biol Chem. 2023 May 30;405(1):55-65. doi: 10.1515/hsz-2023-0175. Print 2024 Jan 29.Biol Chem. 2023.PMID:37246368Review.
Cited by
- Astrocytes: new evidence, new models, new roles.Brazhe A, Verisokin A, Verveyko D, Postnov D.Brazhe A, et al.Biophys Rev. 2023 Oct 18;15(5):1303-1333. doi: 10.1007/s12551-023-01145-7. eCollection 2023 Oct.Biophys Rev. 2023.PMID:37975000Free PMC article.Review.
- ClickArr: a novel, high-throughput assay for evaluating β-arrestin isoform recruitment.French AR, Meqbil YJ, van Rijn RM.French AR, et al.Front Pharmacol. 2023 Nov 7;14:1295518. doi: 10.3389/fphar.2023.1295518. eCollection 2023.Front Pharmacol. 2023.PMID:38027002Free PMC article.
- Single-chain fluorescent integrators for mapping G-protein-coupled receptor agonists.Kroning K, Gannot N, Li X, Putansu A, Zhou G, Sescil J, Shen J, Wilson A, Fiel H, Li P, Wang W.Kroning K, et al.Proc Natl Acad Sci U S A. 2024 Apr 30;121(18):e2307090121. doi: 10.1073/pnas.2307090121. Epub 2024 Apr 22.Proc Natl Acad Sci U S A. 2024.PMID:38648487Free PMC article.
- Protein-Based Tools for Studying Neuromodulation.Wang W.Wang W.ACS Chem Biol. 2024 Apr 19;19(4):788-797. doi: 10.1021/acschembio.4c00037. Epub 2024 Apr 6.ACS Chem Biol. 2024.PMID:38581649Free PMC article.Review.
References
- Tzameli I. GPCRs—pivotal players in metabolism. Trends Endocrinol Metab. 2016;27:597‐599. - PubMed
Publication types
MeSH terms
Substances
Related information
Grants and funding
LinkOut - more resources
Full Text Sources