Cellular adaptations leading to coral fragment attachment on artificial substrates in Acropora millepora (Am-CAM)
- PMID:36319668
- PMCID: PMC9626494
- DOI: 10.1038/s41598-022-23134-8
Cellular adaptations leading to coral fragment attachment on artificial substrates in Acropora millepora (Am-CAM)
Abstract
Reproductive propagation by asexual fragmentation in the reef-building coral Acropora millepora depends on (1) successful attachment to the reef substrate through modification of soft tissues and (2) a permanent bond with skeletal encrustation. Despite decades of research examining asexual propagation in corals, the initial response, cellular reorganisation, and development leading to fragment substrate attachment via a newly formed skeleton has not been documented in its entirety. Here, we establish the first "coral attachment model" for this species ("Am-CAM") by developing novel methods that allow correlation of fluorescence and electron microscopy image data with in vivo microscopic time-lapse imagery. This multi-scale imaging approach identified three distinct phases involved in asexual propagation: (1) the contact response of the coral fragment when contact with the substrate, followed by (2) fragment stabilisation through anchoring by the soft tissue, and (3) formation of a "lappet-like appendage" structure leading to substrate bonding of the tissue for encrustation through the onset of skeletal calcification. In developing Am-CAM, we provide new biological insights that can enable reef researchers, managers and coral restoration practitioners to begin evaluating attachment effectiveness, which is needed to optimise species-substrate compatibility and achieve effective outplanting.
© 2022. The Author(s).
Conflict of interest statement
The authors declare no competing interests.
Figures
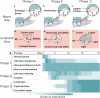
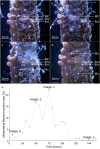
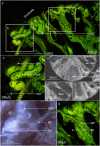
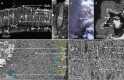
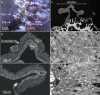
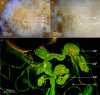
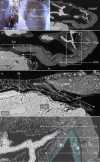
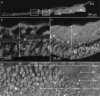
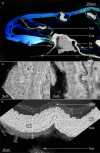
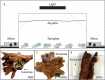
Similar articles
- Regulation of apoptotic mediators reveals dynamic responses to thermal stress in the reef building coral Acropora millepora.Pernice M, Dunn SR, Miard T, Dufour S, Dove S, Hoegh-Guldberg O.Pernice M, et al.PLoS One. 2011 Jan 24;6(1):e16095. doi: 10.1371/journal.pone.0016095.PLoS One. 2011.PMID:21283671Free PMC article.
- Biology and ecology of the hydrocoral millepora on coral reefs.Lewis JB.Lewis JB.Adv Mar Biol. 2006;50:1-55. doi: 10.1016/S0065-2881(05)50001-4.Adv Mar Biol. 2006.PMID:16782450Review.
- Effects of High Dissolved Inorganic and Organic Carbon Availability on the Physiology of the Hard Coral Acropora millepora from the Great Barrier Reef.Meyer FW, Vogel N, Diele K, Kunzmann A, Uthicke S, Wild C.Meyer FW, et al.PLoS One. 2016 Mar 9;11(3):e0149598. doi: 10.1371/journal.pone.0149598. eCollection 2016.PLoS One. 2016.PMID:26959499Free PMC article.
- Regional-scale dominance of non-framework building corals on Caribbean reefs affects carbonate production and future reef growth.Perry CT, Steneck RS, Murphy GN, Kench PS, Edinger EN, Smithers SG, Mumby PJ.Perry CT, et al.Glob Chang Biol. 2015 Mar;21(3):1153-64. doi: 10.1111/gcb.12792. Epub 2014 Dec 23.Glob Chang Biol. 2015.PMID:25537577
- A geological perspective on the degradation and conservation of western Atlantic coral reefs.Kuffner IB, Toth LT.Kuffner IB, et al.Conserv Biol. 2016 Aug;30(4):706-15. doi: 10.1111/cobi.12725. Epub 2016 Apr 29.Conserv Biol. 2016.PMID:27029403Review.
References
- Smith LD, Hughes TP. An experimental assessment of survival, re-attachment and fecundity of coral fragments. J. Exp. Mar. Biol. Ecol. 1999;235:147–164. doi: 10.1016/S0022-0981(98)00178-6. - DOI
- Harriott, V. & Fisk, D. in Proc 6th int coral Reef Symp. 375–379.
- Wallace CC. Reproduction, recruitment and fragmentation in nine sympatric species of the coral genus Acropora. Mar. Biol. 1985;88:217–233. doi: 10.1007/BF00392585. - DOI
- Roniewicz E, Stolarski J. Evolutionary trends in the epithecate scleractinian corals. Acta Palaeontol. Pol. 1999;44:131–166.
- Barnes DJ. The structure and formation of growth-ridges in scleractinian coral skeletons. Proc. R. Soc. Lond. Ser. B Biol. Sci. 1972;182:331–350.
MeSH terms
Related information
LinkOut - more resources
Full Text Sources