DNA-Based Optical Quantification of Ion Transport across Giant Vesicles
- PMID:36222833
- PMCID: PMC9620405
- DOI: 10.1021/acsnano.2c07496
DNA-Based Optical Quantification of Ion Transport across Giant Vesicles
Abstract
Accurate measurements of ion permeability through cellular membranes remains challenging due to the lack of suitable ion-selective probes. Here we use giant unilamellar vesicles (GUVs) as membrane models for the direct visualization of mass translocation at the single-vesicle level. Ion transport is indicated with a fluorescently adjustable DNA-based sensor that accurately detects sub-millimolar variations in K+ concentration. In combination with microfluidics, we employed our DNA-based K+ sensor for extraction of the permeation coefficient of potassium ions. We measured K+ permeability coefficients at least 1 order of magnitude larger than previously reported values from bulk experiments and show that permeation rates across the lipid bilayer increase in the presence of octanol. In addition, an analysis of the K+ flux in different concentration gradients allows us to estimate the complementary H+ flux that dissipates the charge imbalance across the GUV membrane. Subsequently, we show that our sensor can quantify the K+ transport across prototypical cation-selective ion channels, gramicidin A and OmpF, revealing their relative H+/K+ selectivity. Our results show that gramicidin A is much more selective to protons than OmpF with a H+/K+ permeability ratio of ∼104.
Keywords: G-quadruplex; giant unilamellar vesicles; ion channels; ion sensor; ion transport; microfluidics.
Conflict of interest statement
The authors declare no competing financial interest.
Figures
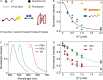
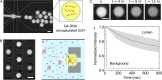
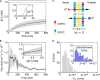
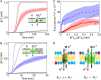
Similar articles
- Imaging Proton Transport in Giant Vesicles through Cyclic Peptide-Polymer Conjugate Nanotube Transmembrane Ion Channels.Binfield JG, Brendel JC, Cameron NR, Eissa AM, Perrier S.Binfield JG, et al.Macromol Rapid Commun. 2018 Oct;39(19):e1700831. doi: 10.1002/marc.201700831. Epub 2018 Feb 16.Macromol Rapid Commun. 2018.PMID:29450934
- Potassium flux through gramicidin ion channels is augmented in vesicles comprised of plasmenylcholine: correlations between gramicidin conformation and function in chemically distinct host bilayer matrices.Chen X, Gross RW.Chen X, et al.Biochemistry. 1995 Jun 6;34(22):7356-64. doi: 10.1021/bi00022a008.Biochemistry. 1995.PMID:7540040
- Proton permeation of lipid bilayers.Deamer DW.Deamer DW.J Bioenerg Biomembr. 1987 Oct;19(5):457-79. doi: 10.1007/BF00770030.J Bioenerg Biomembr. 1987.PMID:2447068Review.
- Proton conduction in gramicidin A and in its dioxolane-linked dimer in different lipid bilayers.Cukierman S, Quigley EP, Crumrine DS.Cukierman S, et al.Biophys J. 1997 Nov;73(5):2489-502. doi: 10.1016/S0006-3495(97)78277-8.Biophys J. 1997.PMID:9370442Free PMC article.
- Elementary Processes and Mechanisms of Interactions of Antimicrobial Peptides with Membranes-Single Giant Unilamellar Vesicle Studies.Hasan M, Yamazaki M.Hasan M, et al.Adv Exp Med Biol. 2019;1117:17-32. doi: 10.1007/978-981-13-3588-4_3.Adv Exp Med Biol. 2019.PMID:30980351Review.
Cited by
- 3D DNA origami pincers that multitask on giant unilamellar vesicles.Zhan P, Yang J, Ding L, Jing X, Hipp K, Nussberger S, Yan H, Liu N.Zhan P, et al.Sci Adv. 2024 Aug 16;10(33):eadn8903. doi: 10.1126/sciadv.adn8903. Epub 2024 Aug 16.Sci Adv. 2024.PMID:39151012Free PMC article.
- Lipid vesicle-based molecular robots.Peng Z, Iwabuchi S, Izumi K, Takiguchi S, Yamaji M, Fujita S, Suzuki H, Kambara F, Fukasawa G, Cooney A, Di Michele L, Elani Y, Matsuura T, Kawano R.Peng Z, et al.Lab Chip. 2024 Feb 27;24(5):996-1029. doi: 10.1039/d3lc00860f.Lab Chip. 2024.PMID:38239102Free PMC article.Review.
References
Publication types
MeSH terms
Substances
Related information
LinkOut - more resources
Full Text Sources