Human cone elongation responses can be explained by photoactivated cone opsin and membrane swelling and osmotic response to phosphate produced by RGS9-catalyzed GTPase
- PMID:36122241
- PMCID: PMC9522364
- DOI: 10.1073/pnas.2202485119
Human cone elongation responses can be explained by photoactivated cone opsin and membrane swelling and osmotic response to phosphate produced by RGS9-catalyzed GTPase
Abstract
Human cone outer segment (COS) length changes in response to stimuli bleaching up to 99% of L- and M-cone opsins were measured with high resolution, phase-resolved optical coherence tomography (OCT). Responses comprised a fast phase (∼5 ms), during which COSs shrink, and two slower phases (1.5 s), during which COSs elongate. The slower components saturated in amplitude (∼425 nm) and initial rate (∼3 nm ms-1) and are well described over the 200-fold bleaching range as the sum of two exponentially rising functions with time constants of 80 to 90 ms (component 1) and 1,000 to 1,250 ms (component 2). Measurements with adaptive optics reflection densitometry revealed component 2 to be linearly related to cone pigment bleaching, and the hypothesis is proposed that it arises from cone opsin and disk membrane swelling triggered by isomerization and rate-limited by chromophore hydrolysis and its reduction to membrane-localized all-trans retinol. The light sensitivity and kinetics of component 1 suggested that the underlying mechanism is an osmotic response to an amplified soluble by-product of phototransduction. The hypotheses that component 1 corresponds to G-protein subunits dissociating from the membrane, metabolites of cyclic guanosine monophosphate (cGMP) hydrolysis, or by-products of activated guanylate cyclase are rejected, while the hypothesis that it corresponds to phosphate produced by regulator of G-protein signaling 9 (RGS9)-catalyzed hydrolysis of guanosine triphosphate (GTP) in G protein-phosphodiesterase complexes was found to be consistent with the results. These results provide a basis for the assessment with optoretinography of phototransduction in individual cone photoreceptors in health and during disease progression and therapeutic interventions.
Keywords: cone photoreceptors; opsin bleaching; optical coherence tomography; optoretinogram; photosensitivity.
Conflict of interest statement
The authors declare no competing interest.
Figures
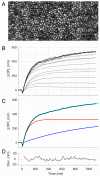
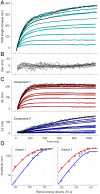
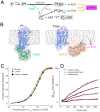
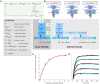
Similar articles
- Effect of 11-cis 13-demethylretinal on phototransduction in bleach-adapted rod and cone photoreceptors.Corson DW, Kefalov VJ, Cornwall MC, Crouch RK.Corson DW, et al.J Gen Physiol. 2000 Aug;116(2):283-97. doi: 10.1085/jgp.116.2.283.J Gen Physiol. 2000.PMID:10919871Free PMC article.
- Occupancy of the chromophore binding site of opsin activates visual transduction in rod photoreceptors.Kefalov VJ, Carter Cornwall M, Crouch RK.Kefalov VJ, et al.J Gen Physiol. 1999 Mar;113(3):491-503. doi: 10.1085/jgp.113.3.491.J Gen Physiol. 1999.PMID:10051522Free PMC article.
- The action of 11-cis-retinol on cone opsins and intact cone photoreceptors.Ala-Laurila P, Cornwall MC, Crouch RK, Kono M.Ala-Laurila P, et al.J Biol Chem. 2009 Jun 12;284(24):16492-16500. doi: 10.1074/jbc.M109.004697. Epub 2009 Apr 22.J Biol Chem. 2009.PMID:19386593Free PMC article.
- A novel role of RGS9: inhibition of retinal guanylyl cyclase.Bondarenko VA, Yu H, Yamazaki RK, Yamazaki A.Bondarenko VA, et al.Mol Cell Biochem. 2002 Jan;230(1-2):125-8.Mol Cell Biochem. 2002.PMID:11952087Review.
- Tuning outer segment Ca2+ homeostasis to phototransduction in rods and cones.Korenbrot JI, Rebrik TI.Korenbrot JI, et al.Adv Exp Med Biol. 2002;514:179-203. doi: 10.1007/978-1-4615-0121-3_11.Adv Exp Med Biol. 2002.PMID:12596922Review.
Cited by
- Optical Assessment of Photoreceptor Function Over the Macula.Ni S, Khan S, Jiménez-Villar A, Pennesi ME, Huang D, Jian Y, Chen S.Ni S, et al.Transl Vis Sci Technol. 2024 Aug 1;13(8):41. doi: 10.1167/tvst.13.8.41.Transl Vis Sci Technol. 2024.PMID:39186303Free PMC article.
- Coarse-scale optoretinography (CoORG) with extended field-of-view for normative characterization.Jiang X, Liu T, Pandiyan VP, Slezak E, Sabesan R.Jiang X, et al.Biomed Opt Express. 2022 Oct 24;13(11):5989-6002. doi: 10.1364/BOE.473475. eCollection 2022 Nov 1.Biomed Opt Express. 2022.PMID:36733759Free PMC article.
- Light-adapted flicker-optoretinography based on raster-scan optical coherence tomography towards clinical translation.Gong Z, Shi Y, Liu J, Sabesan R, Wang RK.Gong Z, et al.Biomed Opt Express. 2024 Sep 26;15(10):6036-6051. doi: 10.1364/BOE.538481. eCollection 2024 Oct 1.Biomed Opt Express. 2024.PMID:39421778Free PMC article.
- Evolution of adaptive optics retinal imaging [Invited].Williams DR, Burns SA, Miller DT, Roorda A.Williams DR, et al.Biomed Opt Express. 2023 Feb 28;14(3):1307-1338. doi: 10.1364/BOE.485371. eCollection 2023 Mar 1.Biomed Opt Express. 2023.PMID:36950228Free PMC article.Review.
- Optical Coherence Tomography Split-Spectrum Amplitude-Decorrelation Optoretinography Detects Early Central Cone Photoreceptor Dysfunction in Retinal Dystrophies.Wongchaisuwat N, Amato A, Yang P, Everett L, Pennesi ME, Huang D, Chen S.Wongchaisuwat N, et al.Transl Vis Sci Technol. 2024 Oct 1;13(10):5. doi: 10.1167/tvst.13.10.5.Transl Vis Sci Technol. 2024.PMID:39361318Free PMC article.
References
- Lim L. S., Mitchell P., Seddon J. M., Holz F. G., Wong T. Y., Age-related macular degeneration. Lancet 379, 1728–1738 (2012). - PubMed
- de Jong P. T., Age-related macular degeneration. N. Engl. J. Med. 355, 1474–1485 (2006). - PubMed
- Drexler W. F., Optical Coherence Tomography (Springer International, 2015).
- Fujimoto J. G. H., Foreword: 25 years of optical coherence tomography. Invest. Ophthalmol. Vis. Sci. 57, 2 (2016). - PubMed
- Roorda A., Williams D. R., The arrangement of the three cone classes in the living human eye. Nature 397, 520–522 (1999). - PubMed
Publication types
MeSH terms
Substances
Related information
Grants and funding
LinkOut - more resources
Full Text Sources