Pharmacological Manipulations of Physiological Arousal and Sleep-Like Slow Waves Modulate Sustained Attention
- PMID:36109167
- PMCID: PMC9637000
- DOI: 10.1523/JNEUROSCI.0836-22.2022
Pharmacological Manipulations of Physiological Arousal and Sleep-Like Slow Waves Modulate Sustained Attention
Abstract
Sustained attention describes our ability to keep a constant focus on a given task. This ability is modulated by our physiological state of arousal. Although lapses of sustained attention have been linked with dysregulations of arousal, the underlying physiological mechanisms remain unclear. An emerging body of work proposes that the intrusion during wakefulness of sleep-like slow waves, a marker of the transition toward sleep, could mechanistically account for attentional lapses. This study aimed to expose, via pharmacological manipulations of the monoamine system, the relationship between the occurrence of sleep-like slow waves and the behavioral consequences of sustained attention failures. In a double-blind, randomized-control trial, 32 healthy human male participants received methylphenidate, atomoxetine, citalopram or placebo during four separate experimental sessions. During each session, electroencephalography (EEG) was used to measure neural activity while participants completed a visual task requiring sustained attention. Methylphenidate, which increases wake-promoting dopamine and noradrenaline across cortical and subcortical areas, improved behavioral performance whereas atomoxetine, which increases dopamine and noradrenaline predominantly over frontal cortices, led to more impulsive responses. Additionally, citalopram, which increases sleep-promoting serotonin, led to more missed trials. Based on EEG recording, citalopram was also associated with an increase in sleep-like slow waves. Importantly, compared with a classical marker of arousal such as α power, only slow waves differentially predicted both misses and faster responses in a region-specific fashion. These results suggest that a decrease in arousal can lead to local sleep intrusions during wakefulness which could be mechanistically linked to impulsivity and sluggishness.SIGNIFICANCE STATEMENT We investigated whether the modulation of attention and arousal could not only share the same neuromodulatory pathways but also rely on similar neuronal mechanisms; for example, the intrusion of sleep-like activity within wakefulness. To do so, we pharmacologically manipulated noradrenaline, dopamine, and serotonin in a four-arm, randomized, placebo-controlled trial and examined the consequences on behavioral and electroencephalography (EEG) indices of attention and arousal. We showed that sleep-like slow waves can predict opposite behavioral signatures: impulsivity and sluggishness. Slow waves may be a candidate mechanism for the occurrence of attentional lapses since the relationship between slow-wave occurrence and performance is region-specific and the consequences of these local sleep intrusions are in line with the cognitive functions carried by the underlying brain regions.
Keywords: arousal; attention; electroencephalography; neuromodulation; sleep; vigilance.
Copyright © 2022 the authors.
Figures
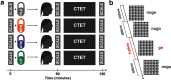
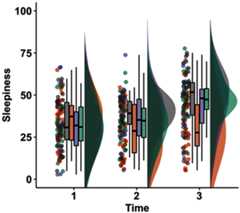
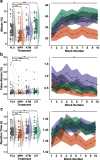
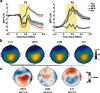
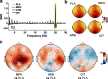
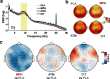
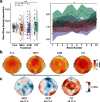
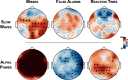
Similar articles
- Predicting lapses of attention with sleep-like slow waves.Andrillon T, Burns A, Mackay T, Windt J, Tsuchiya N.Andrillon T, et al.Nat Commun. 2021 Jun 29;12(1):3657. doi: 10.1038/s41467-021-23890-7.Nat Commun. 2021.PMID:34188023Free PMC article.
- Local experience-dependent changes in the wake EEG after prolonged wakefulness.Hung CS, Sarasso S, Ferrarelli F, Riedner B, Ghilardi MF, Cirelli C, Tononi G.Hung CS, et al.Sleep. 2013 Jan 1;36(1):59-72. doi: 10.5665/sleep.2302.Sleep. 2013.PMID:23288972Free PMC article.
- Dextroamphetamine (but Not Atomoxetine) Induces Reanimation from General Anesthesia: Implications for the Roles of Dopamine and Norepinephrine in Active Emergence.Kenny JD, Taylor NE, Brown EN, Solt K.Kenny JD, et al.PLoS One. 2015 Jul 6;10(7):e0131914. doi: 10.1371/journal.pone.0131914. eCollection 2015.PLoS One. 2015.PMID:26148114Free PMC article.
- [Selective stimulations and lesions of the rat brain nuclei as the models for research of the human sleep pathology mechanisms].Šaponjić J.Šaponjić J.Glas Srp Akad Nauka Med. 2011;(51):85-97.Glas Srp Akad Nauka Med. 2011.PMID:22165729Review.Serbian.
- Assessment of Wakefulness and Brain Arousal Regulation in Psychiatric Research.Sander C, Hensch T, Wittekind DA, Böttger D, Hegerl U.Sander C, et al.Neuropsychobiology. 2015;72(3-4):195-205. doi: 10.1159/000439384. Epub 2016 Feb 23.Neuropsychobiology. 2015.PMID:26901462Review.
Cited by
- Effects of Maternal Separation and Subsequent Stress on Behaviors and Brain Monoamines in Rats.Mavrenkova PV, Khlebnikova NN, Alchinova IB, Demorzhi MS, Shoibonov BB, Karganov MY.Mavrenkova PV, et al.Brain Sci. 2023 Jun 15;13(6):956. doi: 10.3390/brainsci13060956.Brain Sci. 2023.PMID:37371434Free PMC article.
- A systematic review and activation likelihood estimation meta-analysis of fMRI studies on arousing or wake-promoting effects in Buddhist meditation.Chaudhary IS, Shyi GC, Huang ST.Chaudhary IS, et al.Front Psychol. 2023 Oct 27;14:1136983. doi: 10.3389/fpsyg.2023.1136983. eCollection 2023.Front Psychol. 2023.PMID:38022985Free PMC article.
- Illustrations of interactions needed when investigating sleep using a type of AM-PM PM-AM design.Mickes L, Morgan DP, Fuentes Grandón DA, Boogert S, Kazanina N.Mickes L, et al.Psychon Bull Rev. 2023 Dec;30(6):2106-2115. doi: 10.3758/s13423-023-02248-8. Epub 2023 Jun 15.Psychon Bull Rev. 2023.PMID:37322385Free PMC article.Review.
- What Chemsex does to the brain - neural correlates (ERP) regarding decision making, impulsivity and hypersexuality.Schwarz J, Gertzen M, Rabenstein A, Straßburger M, Horstmann A, Pogarell O, Rüther T, Karch S.Schwarz J, et al.Eur Arch Psychiatry Clin Neurosci. 2025 Feb;275(1):227-240. doi: 10.1007/s00406-024-01856-2. Epub 2024 Jul 6.Eur Arch Psychiatry Clin Neurosci. 2025.PMID:38969754Free PMC article.
References
Publication types
MeSH terms
Substances
Related information
LinkOut - more resources
Full Text Sources