Continental flood basalts drive Phanerozoic extinctions
- PMID:36095185
- PMCID: PMC9499591
- DOI: 10.1073/pnas.2120441119
Continental flood basalts drive Phanerozoic extinctions
Abstract
Refinements of the geological timescale driven by the increasing precision and accuracy of radiometric dating have revealed an apparent correlation between large igneous provinces (LIPs) and intervals of Phanerozoic faunal turnover that has been much discussed at a qualitative level. However, the extent to which such correlations are likely to occur by chance has yet to be quantitatively tested, and other kill mechanisms have been suggested for many mass extinctions. Here, we show that the degree of temporal correlation between continental LIPs and faunal turnover in the Phanerozoic is unlikely to occur by chance, suggesting a causal relationship linking extinctions and continental flood basalts. The relationship is stronger for LIPs with higher estimated eruptive rates and for stage boundaries with higher extinction magnitudes. This suggests LIP magma degassing as a primary kill mechanism for mass extinctions and other intervals of faunal turnover, which may be related to [Formula: see text], Cl, and F release. Our results suggest continental LIPs as a major, direct driver of extinctions throughout the Phanerozoic.
Keywords: carbon cycle; mass extinctions; paleontology; volcanology.
Conflict of interest statement
The authors declare no competing interest.
Figures
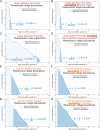
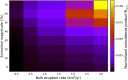
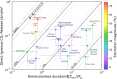
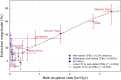
Comment in
- Continental flood basalts do not drive later Phanerozoic extinctions.Henehan MJ, Witts JD.Henehan MJ, et al.Proc Natl Acad Sci U S A. 2023 May 23;120(21):e2303700120. doi: 10.1073/pnas.2303700120. Epub 2023 May 15.Proc Natl Acad Sci U S A. 2023.PMID:37186834Free PMC article.No abstract available.
Similar articles
- Comparing the evidence relevant to impact and flood basalt at times of major mass extinctions.Alvarez W.Alvarez W.Astrobiology. 2003 Spring;3(1):153-61. doi: 10.1089/153110703321632480.Astrobiology. 2003.PMID:12804370
- End-Triassic mass extinction started by intrusive CAMP activity.Davies JHFL, Marzoli A, Bertrand H, Youbi N, Ernesto M, Schaltegger U.Davies JHFL, et al.Nat Commun. 2017 May 31;8:15596. doi: 10.1038/ncomms15596.Nat Commun. 2017.PMID:28561025Free PMC article.
- Compound-specific carbon isotopes from Earth's largest flood basalt eruptions directly linked to the end-Triassic mass extinction.Whiteside JH, Olsen PE, Eglinton T, Brookfield ME, Sambrotto RN.Whiteside JH, et al.Proc Natl Acad Sci U S A. 2010 Apr 13;107(15):6721-5. doi: 10.1073/pnas.1001706107. Epub 2010 Mar 22.Proc Natl Acad Sci U S A. 2010.PMID:20308590Free PMC article.
- Theory and classification of mass extinction causation.Algeo TJ, Shen J.Algeo TJ, et al.Natl Sci Rev. 2023 Sep 8;11(1):nwad237. doi: 10.1093/nsr/nwad237. eCollection 2024 Jan.Natl Sci Rev. 2023.PMID:38116094Free PMC article.Review.
- Extinction from a paleontological perspective.Raup DM.Raup DM.Eur Rev. 1993;1(3):207-16. doi: 10.1017/s1062798700000582.Eur Rev. 1993.PMID:11539838Review.
Cited by
- Reply to Henehan and Witts: Continental flood basalts drive extinctions after the mid-Mesozoic.Green T, Renne PR, Keller CB.Green T, et al.Proc Natl Acad Sci U S A. 2023 May 23;120(21):e2304194120. doi: 10.1073/pnas.2304194120. Epub 2023 May 15.Proc Natl Acad Sci U S A. 2023.PMID:37186831Free PMC article.No abstract available.
- Lineages of Fractal Genera Comprise the 88-Million-Year Steel Evolutionary Spine of the Ecosphere.Zander RH.Zander RH.Plants (Basel). 2024 Jun 5;13(11):1559. doi: 10.3390/plants13111559.Plants (Basel). 2024.PMID:38891367Free PMC article.
- The Cenomanian/Turonian boundary in light of new developments in terrestrial palynology.Galasso F, Heimhofer U, Schneebeli-Hermann E.Galasso F, et al.Sci Rep. 2023 Feb 22;13(1):3074. doi: 10.1038/s41598-023-30072-6.Sci Rep. 2023.PMID:36813802Free PMC article.
- Recurring volcanic winters during the latest Cretaceous: Sulfur and fluorine budgets of Deccan Traps lavas.Callegaro S, Baker DR, Renne PR, Melluso L, Geraki K, Whitehouse MJ, De Min A, Marzoli A.Callegaro S, et al.Sci Adv. 2023 Oct 6;9(40):eadg8284. doi: 10.1126/sciadv.adg8284. Epub 2023 Oct 4.Sci Adv. 2023.PMID:37792933Free PMC article.
- Continental flood basalts do not drive later Phanerozoic extinctions.Henehan MJ, Witts JD.Henehan MJ, et al.Proc Natl Acad Sci U S A. 2023 May 23;120(21):e2303700120. doi: 10.1073/pnas.2303700120. Epub 2023 May 15.Proc Natl Acad Sci U S A. 2023.PMID:37186834Free PMC article.No abstract available.
References
- Coffin M., Eldholm O., eds., “Large igneous provinces” (Joint Oceanographic Institutions/US Scientific Advisory Committee Workshop Report, Cannon Technical Report, The University of Texas at Austin Institute for Geophysics, Austin, TX, 1991).
- Coffin M., Eldholm O., Volcanism and continental break-up: A global compilation of large igneous provinces (Special Publications, Geological Society, London, 1992), vol. 68, pp. 17–30.
- Ernst R. E., Youbi N., How Large Igneous Provinces affect global climate, sometimes cause mass extinctions, and represent natural markers in the geological record. Palaeogeogr. Palaeoclimatol. Palaeoecol. 478, 30–52 (2017).
- Ernst R. E., Bleeker W., Soderlund U., Kerr A. C., Large Igneous Provinces and supercontinents: Toward completing the plate tectonic revolution. Lithos 174, 1–14 (2013).
- Courtillot V. E., Renne P. R., On the ages of flood basalt events. C. R. Geosci. 355, 113–140 (2003).