Identification of carbon dioxide in an exoplanet atmosphere
- PMID:36055338
- PMCID: PMC9946830
- DOI: 10.1038/s41586-022-05269-w
Identification of carbon dioxide in an exoplanet atmosphere
Abstract
Carbon dioxide (CO2) is a key chemical species that is found in a wide range of planetary atmospheres. In the context of exoplanets, CO2 is an indicator of the metal enrichment (that is, elements heavier than helium, also called 'metallicity')1-3, and thus the formation processes of the primary atmospheres of hot gas giants4-6. It is also one of the most promising species to detect in the secondary atmospheres of terrestrial exoplanets7-9. Previous photometric measurements of transiting planets with the Spitzer Space Telescope have given hints of the presence of CO2, but have not yielded definitive detections owing to the lack of unambiguous spectroscopic identification10-12. Here we present the detection of CO2 in the atmosphere of the gas giant exoplanet WASP-39b from transmission spectroscopy observations obtained with JWST as part of the Early Release Science programme13,14. The data used in this study span 3.0-5.5 micrometres in wavelength and show a prominent CO2 absorption feature at 4.3 micrometres (26-sigma significance). The overall spectrum is well matched by one-dimensional, ten-times solar metallicity models that assume radiative-convective-thermochemical equilibrium and have moderate cloud opacity. These models predict that the atmosphere should have water, carbon monoxide and hydrogen sulfide in addition to CO2, but little methane. Furthermore, we also tentatively detect a small absorption feature near 4.0 micrometres that is not reproduced by these models.
© 2022. The Author(s), under exclusive licence to Springer Nature Limited.
Conflict of interest statement
The authors declare no competing interests.
Figures
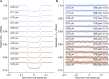
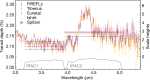
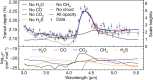
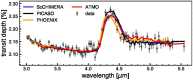
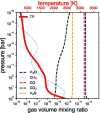
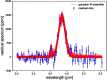
Comment in
- Webb telescope spots CO2 on exoplanet for first time: what it means for finding alien life.Hall S.Hall S.Nature. 2022 Sep;609(7926):229-230. doi: 10.1038/d41586-022-02350-2.Nature. 2022.PMID:36038762No abstract available.
- JWST opens a window on exoplanet skies.Seidel JV, Nielsen LD, Sarkar S.Seidel JV, et al.Nature. 2023 Feb;614(7949):632-633. doi: 10.1038/d41586-023-00394-6.Nature. 2023.PMID:36792896No abstract available.
Similar articles
- Photochemically produced SO2 in the atmosphere of WASP-39b.Tsai SM, Lee EKH, Powell D, Gao P, Zhang X, Moses J, Hébrard E, Venot O, Parmentier V, Jordan S, Hu R, Alam MK, Alderson L, Batalha NM, Bean JL, Benneke B, Bierson CJ, Brady RP, Carone L, Carter AL, Chubb KL, Inglis J, Leconte J, Line M, López-Morales M, Miguel Y, Molaverdikhani K, Rustamkulov Z, Sing DK, Stevenson KB, Wakeford HR, Yang J, Aggarwal K, Baeyens R, Barat S, de Val-Borro M, Daylan T, Fortney JJ, France K, Goyal JM, Grant D, Kirk J, Kreidberg L, Louca A, Moran SE, Mukherjee S, Nasedkin E, Ohno K, Rackham BV, Redfield S, Taylor J, Tremblin P, Visscher C, Wallack NL, Welbanks L, Youngblood A, Ahrer EM, Batalha NE, Behr P, Berta-Thompson ZK, Blecic J, Casewell SL, Crossfield IJM, Crouzet N, Cubillos PE, Decin L, Désert JM, Feinstein AD, Gibson NP, Harrington J, Heng K, Henning T, Kempton EM, Krick J, Lagage PO, Lendl M, Lothringer JD, Mansfield M, Mayne NJ, Mikal-Evans T, Palle E, Schlawin E, Shorttle O, Wheatley PJ, Yurchenko SN.Tsai SM, et al.Nature. 2023 May;617(7961):483-487. doi: 10.1038/s41586-023-05902-2. Epub 2023 Apr 26.Nature. 2023.PMID:37100917Free PMC article.
- Early Release Science of the exoplanet WASP-39b with JWST NIRCam.Ahrer EM, Stevenson KB, Mansfield M, Moran SE, Brande J, Morello G, Murray CA, Nikolov NK, Petit Dit de la Roche DJM, Schlawin E, Wheatley PJ, Zieba S, Batalha NE, Damiano M, Goyal JM, Lendl M, Lothringer JD, Mukherjee S, Ohno K, Batalha NM, Battley MP, Bean JL, Beatty TG, Benneke B, Berta-Thompson ZK, Carter AL, Cubillos PE, Daylan T, Espinoza N, Gao P, Gibson NP, Gill S, Harrington J, Hu R, Kreidberg L, Lewis NK, Line MR, López-Morales M, Parmentier V, Powell DK, Sing DK, Tsai SM, Wakeford HR, Welbanks L, Alam MK, Alderson L, Allen NH, Anderson DR, Barstow JK, Bayliss D, Bell TJ, Blecic J, Bryant EM, Burleigh MR, Carone L, Casewell SL, Changeat Q, Chubb KL, Crossfield IJM, Crouzet N, Decin L, Désert JM, Feinstein AD, Flagg L, Fortney JJ, Gizis JE, Heng K, Iro N, Kempton EM, Kendrew S, Kirk J, Knutson HA, Komacek TD, Lagage PO, Leconte J, Lustig-Yaeger J, MacDonald RJ, Mancini L, May EM, Mayne NJ, Miguel Y, Mikal-Evans T, Molaverdikhani K, Palle E, Piaulet C, Rackham BV, Redfield S, Rogers LK, Roy PA, Rustamkulov Z, Shkolnik EL, Sotzen KS, Taylor J, Tremblin P, Tucker GS, Turner JD, de Val-Borro M, Venot O, Zhang X.Ahrer EM, et al.Nature. 2023 Feb;614(7949):653-658. doi: 10.1038/s41586-022-05590-4. Epub 2023 Jan 9.Nature. 2023.PMID:36623551Free PMC article.
- Early Release Science of the exoplanet WASP-39b with JWST NIRSpec PRISM.Rustamkulov Z, Sing DK, Mukherjee S, May EM, Kirk J, Schlawin E, Line MR, Piaulet C, Carter AL, Batalha NE, Goyal JM, López-Morales M, Lothringer JD, MacDonald RJ, Moran SE, Stevenson KB, Wakeford HR, Espinoza N, Bean JL, Batalha NM, Benneke B, Berta-Thompson ZK, Crossfield IJM, Gao P, Kreidberg L, Powell DK, Cubillos PE, Gibson NP, Leconte J, Molaverdikhani K, Nikolov NK, Parmentier V, Roy P, Taylor J, Turner JD, Wheatley PJ, Aggarwal K, Ahrer E, Alam MK, Alderson L, Allen NH, Banerjee A, Barat S, Barrado D, Barstow JK, Bell TJ, Blecic J, Brande J, Casewell S, Changeat Q, Chubb KL, Crouzet N, Daylan T, Decin L, Désert J, Mikal-Evans T, Feinstein AD, Flagg L, Fortney JJ, Harrington J, Heng K, Hong Y, Hu R, Iro N, Kataria T, Kempton EM, Krick J, Lendl M, Lillo-Box J, Louca A, Lustig-Yaeger J, Mancini L, Mansfield M, Mayne NJ, Miguel Y, Morello G, Ohno K, Palle E, Petit Dit de la Roche DJM, Rackham BV, Radica M, Ramos-Rosado L, Redfield S, Rogers LK, Shkolnik EL, Southworth J, Teske J, Tremblin P, Tucker GS, Venot O, Waalkes WC, Welbanks L, Zhang X, Zieba S.Rustamkulov Z, et al.Nature. 2023 Feb;614(7949):659-663. doi: 10.1038/s41586-022-05677-y. Epub 2023 Jan 9.Nature. 2023.PMID:36623548Free PMC article.
- M stars as targets for terrestrial exoplanet searches and biosignature detection.Scalo J, Kaltenegger L, Segura A, Fridlund M, Ribas I, Kulikov YN, Grenfell JL, Rauer H, Odert P, Leitzinger M, Selsis F, Khodachenko ML, Eiroa C, Kasting J, Lammer H.Scalo J, et al.Astrobiology. 2007 Feb;7(1):85-166. doi: 10.1089/ast.2006.0125.Astrobiology. 2007.PMID:17407405Review.
- A Review of Possible Planetary Atmospheres in the TRAPPIST-1 System.Turbet M, Bolmont E, Bourrier V, Demory BO, Leconte J, Owen J, Wolf ET.Turbet M, et al.Space Sci Rev. 2020;216(5):100. doi: 10.1007/s11214-020-00719-1. Epub 2020 Jul 23.Space Sci Rev. 2020.PMID:32764836Free PMC article.Review.
Cited by
- Photochemically produced SO2 in the atmosphere of WASP-39b.Tsai SM, Lee EKH, Powell D, Gao P, Zhang X, Moses J, Hébrard E, Venot O, Parmentier V, Jordan S, Hu R, Alam MK, Alderson L, Batalha NM, Bean JL, Benneke B, Bierson CJ, Brady RP, Carone L, Carter AL, Chubb KL, Inglis J, Leconte J, Line M, López-Morales M, Miguel Y, Molaverdikhani K, Rustamkulov Z, Sing DK, Stevenson KB, Wakeford HR, Yang J, Aggarwal K, Baeyens R, Barat S, de Val-Borro M, Daylan T, Fortney JJ, France K, Goyal JM, Grant D, Kirk J, Kreidberg L, Louca A, Moran SE, Mukherjee S, Nasedkin E, Ohno K, Rackham BV, Redfield S, Taylor J, Tremblin P, Visscher C, Wallack NL, Welbanks L, Youngblood A, Ahrer EM, Batalha NE, Behr P, Berta-Thompson ZK, Blecic J, Casewell SL, Crossfield IJM, Crouzet N, Cubillos PE, Decin L, Désert JM, Feinstein AD, Gibson NP, Harrington J, Heng K, Henning T, Kempton EM, Krick J, Lagage PO, Lendl M, Lothringer JD, Mansfield M, Mayne NJ, Mikal-Evans T, Palle E, Schlawin E, Shorttle O, Wheatley PJ, Yurchenko SN.Tsai SM, et al.Nature. 2023 May;617(7961):483-487. doi: 10.1038/s41586-023-05902-2. Epub 2023 Apr 26.Nature. 2023.PMID:37100917Free PMC article.
- Can Isotopologues Be Used as Biosignature Gases in Exoplanet Atmospheres?Glidden A, Seager S, Petkowski JJ, Ono S.Glidden A, et al.Life (Basel). 2023 Dec 11;13(12):2325. doi: 10.3390/life13122325.Life (Basel). 2023.PMID:38137926Free PMC article.Review.
- Sulfur dioxide in the mid-infrared transmission spectrum of WASP-39b.Powell D, Feinstein AD, Lee EKH, Zhang M, Tsai SM, Taylor J, Kirk J, Bell T, Barstow JK, Gao P, Bean JL, Blecic J, Chubb KL, Crossfield IJM, Jordan S, Kitzmann D, Moran SE, Morello G, Moses JI, Welbanks L, Yang J, Zhang X, Ahrer EM, Bello-Arufe A, Brande J, Casewell SL, Crouzet N, Cubillos PE, Demory BO, Dyrek A, Flagg L, Hu R, Inglis J, Jones KD, Kreidberg L, López-Morales M, Lagage PO, Meier Valdés EA, Miguel Y, Parmentier V, Piette AAA, Rackham BV, Radica M, Redfield S, Stevenson KB, Wakeford HR, Aggarwal K, Alam MK, Batalha NM, Batalha NE, Benneke B, Berta-Thompson ZK, Brady RP, Caceres C, Carter AL, Désert JM, Harrington J, Iro N, Line MR, Lothringer JD, MacDonald RJ, Mancini L, Molaverdikhani K, Mukherjee S, Nixon MC, Oza AV, Palle E, Rustamkulov Z, Sing DK, Steinrueck ME, Venot O, Wheatley PJ, Yurchenko SN.Powell D, et al.Nature. 2024 Feb;626(8001):979-983. doi: 10.1038/s41586-024-07040-9. Epub 2024 Jan 17.Nature. 2024.PMID:38232945Free PMC article.
- Direct observation of coherence transfer and rotational-to-vibrational energy exchange in optically centrifuged CO2 super-rotors.Chen TY, Steinmetz SA, Patterson BD, Jasper AW, Kliewer CJ.Chen TY, et al.Nat Commun. 2023 Jun 3;14(1):3227. doi: 10.1038/s41467-023-38873-z.Nat Commun. 2023.PMID:37270647Free PMC article.
- Experimental Polarizability Transition Moments of CO2 for Excited Vibrational States.Álvarez C, Tejeda G, Fernández JM.Álvarez C, et al.Molecules. 2024 Oct 29;29(21):5103. doi: 10.3390/molecules29215103.Molecules. 2024.PMID:39519746Free PMC article.
References
- Lodders K, Fegley B. Atmospheric chemistry in giant planets, brown dwarfs, and low-mass dwarf stars. I. Carbon, nitrogen, and oxygen. Icarus. 2002;155:393–424. doi: 10.1006/icar.2001.6740. - DOI
- Zahnle K, Marley MS, Freedman RS, Lodders K, Fortney JJ. Atmospheric sulfur photochemistry on hot Jupiters. Astrophys. J. Lett. 2009;701:L20–L24. doi: 10.1088/0004-637X/701/1/L20. - DOI
- Pollack JB, et al. Formation of the giant planets by concurrent accretion of solids and gas. Icarus. 1996;124:62–85. doi: 10.1006/icar.1996.0190. - DOI
- Fortney JJ, et al. A framework for characterizing the atmospheres of low-mass low-density transiting planets. Astrophys. J. 2013;775:80. doi: 10.1088/0004-637X/775/1/80. - DOI