Cooperativity and Interdependency between RNA Structure and RNA-RNA Interactions
- PMID:34940761
- PMCID: PMC8704770
- DOI: 10.3390/ncrna7040081
Cooperativity and Interdependency between RNA Structure and RNA-RNA Interactions
Abstract
Complex RNA-RNA interactions are increasingly known to play key roles in numerous biological processes from gene expression control to ribonucleoprotein granule formation. By contrast, the nature of these interactions and characteristics of their interfaces, especially those that involve partially or wholly structured RNAs, remain elusive. Herein, we discuss different modalities of RNA-RNA interactions with an emphasis on those that depend on secondary, tertiary, or quaternary structure. We dissect recently structurally elucidated RNA-RNA complexes including RNA triplexes, riboswitches, ribozymes, and reverse transcription complexes. These analyses highlight a reciprocal relationship that intimately links RNA structure formation with RNA-RNA interactions. The interactions not only shape and sculpt RNA structures but also are enabled and modulated by the structures they create. Understanding this two-way relationship between RNA structure and interactions provides mechanistic insights into the expanding repertoire of noncoding RNA functions, and may inform the design of novel therapeutics that target RNA structures or interactions.
Keywords: RNA; RNA structure; RNA–RNA interactions; RNase P; T-box; base pair; base stacking; riboswitch; ribozyme; tRNA.
Conflict of interest statement
The authors declare no conflict of interest.
Figures
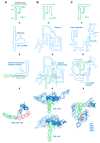
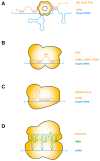
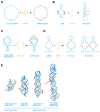
Similar articles
- Unboxing the T-box riboswitches-A glimpse into multivalent and multimodal RNA-RNA interactions.Zhang J.Zhang J.Wiley Interdiscip Rev RNA. 2020 Nov;11(6):e1600. doi: 10.1002/wrna.1600. Epub 2020 Jul 6.Wiley Interdiscip Rev RNA. 2020.PMID:32633085Free PMC article.Review.
- Trying on tRNA for Size: RNase P and the T-box Riboswitch as Molecular Rulers.Zhang J, Ferré-DAmaré AR.Zhang J, et al.Biomolecules. 2016 Apr 1;6(2):18. doi: 10.3390/biom6020018.Biomolecules. 2016.PMID:27043647Free PMC article.Review.
- The dynamic nature of RNA as key to understanding riboswitch mechanisms.Haller A, Soulière MF, Micura R.Haller A, et al.Acc Chem Res. 2011 Dec 20;44(12):1339-48. doi: 10.1021/ar200035g. Epub 2011 Jun 16.Acc Chem Res. 2011.PMID:21678902
- An evolving tale of two interacting RNAs-themes and variations of the T-box riboswitch mechanism.Suddala KC, Zhang J.Suddala KC, et al.IUBMB Life. 2019 Aug;71(8):1167-1180. doi: 10.1002/iub.2098. Epub 2019 Jun 17.IUBMB Life. 2019.PMID:31206978Free PMC article.Review.
- Metabolite-binding ribozymes.Ramesh A, Winkler WC.Ramesh A, et al.Biochim Biophys Acta. 2014 Oct;1839(10):989-994. doi: 10.1016/j.bbagrm.2014.04.015. Epub 2014 Apr 24.Biochim Biophys Acta. 2014.PMID:24769284Review.
Cited by
- Discerning the Prospects of miRNAs as a Multi-Target Therapeutic and Diagnostic for Alzheimer's Disease.Bhatnagar D, Ladhe S, Kumar D.Bhatnagar D, et al.Mol Neurobiol. 2023 Oct;60(10):5954-5974. doi: 10.1007/s12035-023-03446-0. Epub 2023 Jun 29.Mol Neurobiol. 2023.PMID:37386272Review.
- Lineage-specific insertions in T-box riboswitches modulate antibiotic binding and action.Giarimoglou N, Kouvela A, Patsi I, Zhang J, Stamatopoulou V, Stathopoulos C.Giarimoglou N, et al.Nucleic Acids Res. 2022 Jun 10;50(10):5834-5849. doi: 10.1093/nar/gkac359.Nucleic Acids Res. 2022.PMID:35580054Free PMC article.
- A Newly Identified Peripheral Duplex Anchors and Stabilizes the MALAT1 Triplex.Mwangi MN, Yonkunas MJ, Ageeli AA, McGovern-Gooch KR, Yilmaz S, Baird NJ.Mwangi MN, et al.Biochemistry. 2024 Sep 17;63(18):2280-2292. doi: 10.1021/acs.biochem.4c00156. Epub 2024 Aug 27.Biochemistry. 2024.PMID:39190685Free PMC article.
- Unique Features of Satellite DNA Transcription in Different Tissues ofCaenorhabditis elegans.Subirana JA, Messeguer X.Subirana JA, et al.Int J Mol Sci. 2023 Feb 3;24(3):2970. doi: 10.3390/ijms24032970.Int J Mol Sci. 2023.PMID:36769294Free PMC article.
- A model industrial workhorse: Bacillus subtilis strain 168 and its genome after a quarter of a century.Bremer E, Calteau A, Danchin A, Harwood C, Helmann JD, Médigue C, Palsson BO, Sekowska A, Vallenet D, Zuniga A, Zuniga C.Bremer E, et al.Microb Biotechnol. 2023 Jun;16(6):1203-1231. doi: 10.1111/1751-7915.14257. Epub 2023 Apr 1.Microb Biotechnol. 2023.PMID:37002859Free PMC article.Review.
References
Publication types
Grants and funding
LinkOut - more resources
Full Text Sources