Challenges and Strategies of Chemical Analysis of Drugs of Abuse and Explosives by Mass Spectrometry
- PMID:33537286
- PMCID: PMC7847941
- DOI: 10.3389/fchem.2020.598487
Challenges and Strategies of Chemical Analysis of Drugs of Abuse and Explosives by Mass Spectrometry
Abstract
In analytical science, mass spectrometry (MS) is known as a "gold analytical tool" because of its unique character of providing the direct molecular structural information of the relevant analyte molecules. Therefore, MS technique has widely been used in all branches of chemistry along with in proteomics, metabolomics, genomics, lipidomics, environmental monitoring etc. Mass spectrometry-based methods are very much needed for fast and reliable detection and quantification of drugs of abuse and explosives in order to provide fingerprint information for criminal investigation as well as for public security and safety at public places, respectively. Most of the compounds exist as their neutral form in nature except proteins, peptides, nucleic acids that are in ionic forms intrinsically. In MS, ion source is the heart of the MS that is used for ionizing the electrically neutral molecules. Performance of MS in terms of sensitivity and selectivity depends mainly on the efficiency of the ionization source. Accordingly, much attention has been paid to develop efficient ion sources for a wide range of compounds. Unfortunately, none of the commercial ion sources can be used for ionization of different types of compounds. Moreover, in MS, analyte molecules must be released into the gaseous phase and then ionize by using a suitable ion source for detection/quantification. Under these circumstances, fabrication of new ambient ion source and ultrasonic cutter blade-based non-thermal and thermal desorption methods have been taken into account. In this paper, challenges and strategies of mass spectrometry analysis of the drugs of abuse and explosives through fabrication of ambient ionization sources and new desorption methods for non-volatile compounds have been described. We will focus the literature progress mostly in the last decade and present our views for the future study.
Keywords: ambient ionization source; drugs of abuse; explosives; headspace method; hollow cathode discharge ionization; mechanism of ionization and desorption; non-thermal desorption; tribological effect.
Copyright © 2021 Habib, Bi, Hong and Wen.
Conflict of interest statement
HH was employed by company China Innovation Instrument Co., Ltd, Ningbo 315000, Zhejiang, China. The remaining authors declare that the research was conducted in the absence of any commercial or financial relationships that could be construed as a potential conflict of interest.
Figures
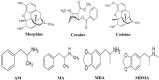
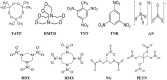
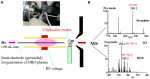
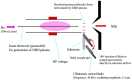
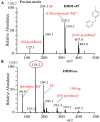
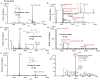
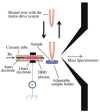
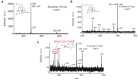
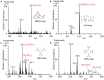
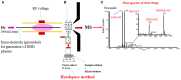
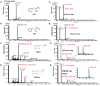
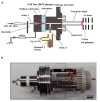
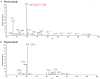
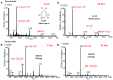
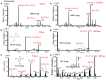
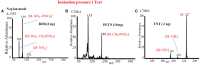
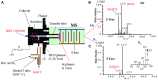
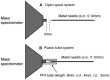
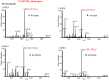
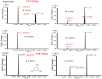
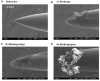
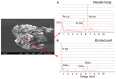
Similar articles
- Ultra-trace level detection of nonvolatile compounds studied by ultrasonic cutter blade coupled with dielectric barrier discharge ionization-mass spectrometry.Bi L, Habib A, Chen L, Xu T, Wen L.Bi L, et al.Talanta. 2021 Jan 15;222:121673. doi: 10.1016/j.talanta.2020.121673. Epub 2020 Sep 18.Talanta. 2021.PMID:33167282
- Hollow Cathode Discharge Ionization Mass Spectrometry: Detection, Quantification and Gas Phase Ion-Molecule Reactions of Explosives and Related Compounds.Hong H, Habib A, Bi L, Qais DS, Wen L.Hong H, et al.Crit Rev Anal Chem. 2024 Jul;54(1):148-174. doi: 10.1080/10408347.2022.2067467. Epub 2022 Apr 25.Crit Rev Anal Chem. 2024.PMID:35467991Review.
- Dual Ambient Sampling Hollow Cathode Discharge Ionization-Mass Spectrometry System: An Approach for Detecting Explosives and Drugs of Abuse at Trace to Ultratrace Levels.Shi L, Habib A, Bi L, Wen L.Shi L, et al.J Am Soc Mass Spectrom. 2024 Sep 4;35(9):2209-2221. doi: 10.1021/jasms.4c00248. Epub 2024 Aug 20.J Am Soc Mass Spectrom. 2024.PMID:39164201
- Desorption electrospray ionization of explosives on surfaces: sensitivity and selectivity enhancement by reactive desorption electrospray ionization.Cotte-Rodríguez I, Takáts Z, Talaty N, Chen H, Cooks RG.Cotte-Rodríguez I, et al.Anal Chem. 2005 Nov 1;77(21):6755-64. doi: 10.1021/ac050995+.Anal Chem. 2005.PMID:16255571
- Ambient Ionization Mass Spectrometry: Application and Prospective.Shi L, Habib A, Bi L, Hong H, Begum R, Wen L.Shi L, et al.Crit Rev Anal Chem. 2024;54(6):1584-1633. doi: 10.1080/10408347.2022.2124840. Epub 2022 Oct 7.Crit Rev Anal Chem. 2024.PMID:36206159Review.
Cited by
- Interpol review of the analysis and detection of explosives and explosives residues.Klapec DJ, Czarnopys G, Pannuto J.Klapec DJ, et al.Forensic Sci Int Synerg. 2023 Jan 13;6:100298. doi: 10.1016/j.fsisyn.2022.100298. eCollection 2023.Forensic Sci Int Synerg. 2023.PMID:36685733Free PMC article.Review.No abstract available.
- Direct Mass Spectrometric Analysis of Semivolatiles Derived from Real Samples at Atmospheric Pressure.Huang DY, Tsai JJ, Chen YC.Huang DY, et al.ACS Omega. 2022 Mar 18;7(12):10255-10261. doi: 10.1021/acsomega.1c06869. eCollection 2022 Mar 29.ACS Omega. 2022.PMID:35382327Free PMC article.
References
- Abdelhamid H. N. (2019b). Nanoparticles assisted laser desorption/ionization mass spectrometry, in Chapter 23: Handbook of Smart Materials in Analytical Chemistry (Chichester: John Wiley & Sons, Ltd; ), 729–755.
- Abdelhamid H. N., Wu H.-F. (2019a). Graphene and its derivatives as platforms for MALDI-MS, in Handbook of Graphene 2, Vol 2: Physics, Chemistry and Biology, eds Tobias S. (Beverly, MA: Scrivener Publishing; ).
Publication types
LinkOut - more resources
Full Text Sources
Other Literature Sources