The Role of Meteorite Impacts in the Origin of Life
- PMID:32876492
- PMCID: PMC7499892
- DOI: 10.1089/ast.2019.2203
The Role of Meteorite Impacts in the Origin of Life
Abstract
The conditions, timing, and setting for the origin of life on Earth and whether life exists elsewhere in our solar system and beyond represent some of the most fundamental scientific questions of our time. Although the bombardment of planets and satellites by asteroids and comets has long been viewed as a destructive process that would have presented a barrier to the emergence of life and frustrated or extinguished life, we provide a comprehensive synthesis of data and observations on the beneficial role of impacts in a wide range of prebiotic and biological processes. In the context of previously proposed environments for the origin of life on Earth, we discuss how meteorite impacts can generate both subaerial and submarine hydrothermal vents, abundant hydrothermal-sedimentary settings, and impact analogues for volcanic pumice rafts and splash pools. Impact events can also deliver and/or generate many of the necessary chemical ingredients for life and catalytic substrates such as clays as well. The role that impact cratering plays in fracturing planetary crusts and its effects on deep subsurface habitats for life are also discussed. In summary, we propose that meteorite impact events are a fundamental geobiological process in planetary evolution that played an important role in the origin of life on Earth. We conclude with the recommendation that impact craters should be considered prime sites in the search for evidence of past life on Mars. Furthermore, unlike other geological processes such as volcanism or plate tectonics, impact cratering is ubiquitous on planetary bodies throughout the Universe and is independent of size, composition, and distance from the host star. Impact events thus provide a mechanism with the potential to generate habitable planets, moons, and asteroids throughout the Solar System and beyond.
Keywords: Crater lakes; Geobiology; Hadean environment; Hydrothermal systems; Impact craters; Lithophytic habitats; Origin of life.
Figures
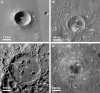
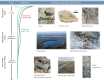
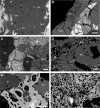
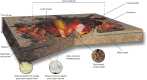
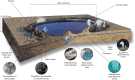
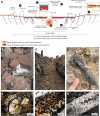
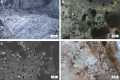
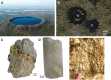
Similar articles
- A symbiotic view of the origin of life at hydrothermal impact crater-lakes.Chatterjee S.Chatterjee S.Phys Chem Chem Phys. 2016 Jul 27;18(30):20033-46. doi: 10.1039/c6cp00550k.Phys Chem Chem Phys. 2016.PMID:27126878
- The origin and emergence of life under impact bombardment.Cockell CS.Cockell CS.Philos Trans R Soc Lond B Biol Sci. 2006 Oct 29;361(1474):1845-55; discussion 1856. doi: 10.1098/rstb.2006.1908.Philos Trans R Soc Lond B Biol Sci. 2006.PMID:17008223Free PMC article.
- Shatter cones: (Mis)understood?Osinski GR, Ferrière L.Osinski GR, et al.Sci Adv. 2016 Aug 5;2(8):e1600616. doi: 10.1126/sciadv.1600616. eCollection 2016 Aug.Sci Adv. 2016.PMID:27532050Free PMC article.
- Microbial Life in Impact Craters.Cockell CS, Osinski G, Sapers H, Pontefract A, Parnell J.Cockell CS, et al.Curr Issues Mol Biol. 2020;38:75-102. doi: 10.21775/cimb.038.075. Epub 2020 Jan 22.Curr Issues Mol Biol. 2020.PMID:31967577Review.
- Are Impact Craters and Extinction Episodes Periodic? Implications for Planetary Science and Astrobiology.Rampino MR, Prokoph A.Rampino MR, et al.Astrobiology. 2020 Sep;20(9):1097-1108. doi: 10.1089/ast.2019.2043. Epub 2020 Aug 28.Astrobiology. 2020.PMID:32865423Review.
Cited by
- Macrobiont: Cradle for the Origin of Life and Creation of a Biosphere.Clark BC, Kolb VM.Clark BC, et al.Life (Basel). 2020 Nov 12;10(11):278. doi: 10.3390/life10110278.Life (Basel). 2020.PMID:33198206Free PMC article.
- Setting the geological scene for the origin of life and continuing open questions about its emergence.Westall F, Brack A, Fairén AG, Schulte MD.Westall F, et al.Front Astron Space Sci. 2023 Jan 5;9:1095701. doi: 10.3389/fspas.2022.1095701.Front Astron Space Sci. 2023.PMID:38274407Free PMC article.
- Spontaneous Emergence of Transient Chirality in Closed, Reversible Frank-like Deterministic Models.Buhse T, Micheau JC.Buhse T, et al.Orig Life Evol Biosph. 2022 Sep;52(1-3):3-20. doi: 10.1007/s11084-022-09621-y. Epub 2022 Jun 10.Orig Life Evol Biosph. 2022.PMID:35680768
- Information Gradient among Nucleotide Sequences of Essential RNAs from an Evolutionary Perspective.Khalfallah HB, Jelassi M, Rissaoui H, Barchouchi M, Baraille C, Gardes J, Demongeot J.Khalfallah HB, et al.Int J Mol Sci. 2024 Jul 9;25(14):7521. doi: 10.3390/ijms25147521.Int J Mol Sci. 2024.PMID:39062761Free PMC article.
- Synthesis of prebiotic organics from CO2 by catalysis with meteoritic and volcanic particles.Peters S, Semenov DA, Hochleitner R, Trapp O.Peters S, et al.Sci Rep. 2023 May 25;13(1):6843. doi: 10.1038/s41598-023-33741-8.Sci Rep. 2023.PMID:37231067Free PMC article.
References
- Abramov O. and Kring D.A. (2004) Numerical modeling of an impact-induced hydrothermal system at the Sudbury Crater. J Geophys Res 109, doi:10.1029/2003JE002213 - DOI
- Abramov O. and Kring D.A. (2007) Numerical modeling of impact-induced hydrothermal activity at the Chicxulub Crater. Meteorit Planet Sci 42:93–112
- Abramov O. and Mojzsis S.J. (2009) Microbial habitability of the Hadean Earth during the Late Heavy Bombardment. Nature 459:419–422 - PubMed
- Acuña M.H., Connerney J.E.P., Ness N.F., Lin R.P., Mitchell D., Carlson C.W., McFadden J., Anderson K.A., Rème H., Mazelle C., Vignes D., Wasilewski P., Cloutier P. (1999) Global distribution of crustal magnetization discovered by the Mars Global Surveyor MAG/ER experiment. Science 284:790–793 - PubMed
- Altwegg K., Balsiger H., Bar-Nun A., Berthelier J.-J., Bieler A., Bochsler P., Briois C., Calmonte U., Combi M.R., Cottin H., De Keyser J., Dhooghe F., Fiethe B., Fuselier S.A., Gasc S., Gombosi T.I., Hansen K.C., Haessig M., Jäckel A., Kopp E., Korth A., Le Roy L., Mall U., Marty B., Mousis O., Owen T., Rème H., Rubin M., Sémon T., Tzou C.-Y., Hunter Waite J., and Wurz P. (2016) Prebiotic chemicals—amino acid and phosphorus—in the coma of comet 67P/Churyumov-Gerasimenko. Sci Adv 2, doi:10.1126/sciadv.1600285 - DOI - PMC - PubMed
Publication types
MeSH terms
Related information
LinkOut - more resources
Full Text Sources
Miscellaneous