Routes to global glaciation
- PMID:32831615
- PMCID: PMC7426044
- DOI: 10.1098/rspa.2020.0303
Routes to global glaciation
Abstract
Theory and observation suggest that Earth and Earth-like planets can undergo runaway low-latitude glaciation when changes in solar heating or in the carbon cycle exceed a critical threshold. Here, we use a simple dynamical-system representation of the ice-albedo feedback and the carbonate-silicate cycle to show that glaciation is also triggered when solar heating changes faster than a critical rate. Such 'rate-induced glaciations' remain accessible far from the outer edge of the habitable zone, because the warm climate state retains long-term stability. In contrast, glaciations induced by changes in the carbon cycle require the warm climate state to become unstable, constraining the kinds of perturbations that could have caused global glaciation in Earth's past. We show that glaciations can occur when Earth's climate transitions between two warm stable states; this property of the Earth system could help explain why major events in the development of life have been accompanied by glaciations.
Keywords: Earth system; dynamical systems; glaciation; snowball Earth; tipping points.
© 2020 The Author(s).
Conflict of interest statement
We declare that we have no competing interests.
Figures
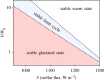
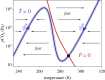
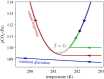
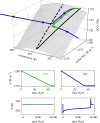
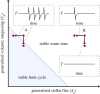
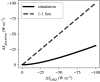
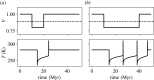
Similar articles
- Impact-induced initiation of Snowball Earth: A model study.Fu M, Abbot DS, Koeberl C, Fedorov A.Fu M, et al.Sci Adv. 2024 Feb 9;10(6):eadk5489. doi: 10.1126/sciadv.adk5489. Epub 2024 Feb 9.Sci Adv. 2024.PMID:38335287Free PMC article.
- Neoproterozoic syn-glacial carbonate precipitation and implications for a snowball Earth.Hood AVS, Penman DE, Lechte MA, Wallace MW, Giddings JA, Planavsky NJ.Hood AVS, et al.Geobiology. 2022 Mar;20(2):175-193. doi: 10.1111/gbi.12470. Epub 2021 Sep 16.Geobiology. 2022.PMID:34528380
- Susceptibility of the early Earth to irreversible glaciation caused by carbon dioxide clouds.Caldeira K, Kasting JF.Caldeira K, et al.Nature. 1992 Sep 17;359:226-8. doi: 10.1038/359226a0.Nature. 1992.PMID:11540934
- M stars as targets for terrestrial exoplanet searches and biosignature detection.Scalo J, Kaltenegger L, Segura A, Fridlund M, Ribas I, Kulikov YN, Grenfell JL, Rauer H, Odert P, Leitzinger M, Selsis F, Khodachenko ML, Eiroa C, Kasting J, Lammer H.Scalo J, et al.Astrobiology. 2007 Feb;7(1):85-166. doi: 10.1089/ast.2006.0125.Astrobiology. 2007.PMID:17407405Review.
- Beyond the principle of plentitude: a review of terrestrial planet habitability.Gaidos E, Deschenes B, Dundon L, Fagan K, Menviel-Hessler L, Moskovitz N, Workman M.Gaidos E, et al.Astrobiology. 2005 Apr;5(2):100-26. doi: 10.1089/ast.2005.5.100.Astrobiology. 2005.PMID:15815163Review.
Cited by
- Impact-induced initiation of Snowball Earth: A model study.Fu M, Abbot DS, Koeberl C, Fedorov A.Fu M, et al.Sci Adv. 2024 Feb 9;10(6):eadk5489. doi: 10.1126/sciadv.adk5489. Epub 2024 Feb 9.Sci Adv. 2024.PMID:38335287Free PMC article.
- Rate-induced collapse in evolutionary systems.Arnscheidt CW, Rothman DH.Arnscheidt CW, et al.J R Soc Interface. 2022 Jun;19(191):20220182. doi: 10.1098/rsif.2022.0182. Epub 2022 Jun 1.J R Soc Interface. 2022.PMID:35642430Free PMC article.
References
- Kirschvink JL. 1992. Late Proterozoic low-latitude global glaciation: the snowball earth. In The Proterozoic biosphere: a multidisciplinary study (eds JW Schopf, C Klein, D Des Marais), pp. 51–52. Cambridge, UK: Cambridge University Press.
- Evans D, Beukes NJ, Kirschvink JL. 1997. Low-latitude glaciation in the Palaeoproterozoic era. Nature 386, 262–266. (10.1038/386262a0) - DOI
- Pierrehumbert R, Abbot D, Voigt A, Koll D. 2011. Climate of the neoproterozoic. Annu. Rev. Earth Planet. Sci. 39, 417–460. (10.1146/annurev-earth-040809-152447) - DOI
Associated data
LinkOut - more resources
Full Text Sources
Miscellaneous