In vivo mapping of a GPCR interactome using knockin mice
- PMID:32457152
- PMCID: PMC7293596
- DOI: 10.1073/pnas.1917906117
In vivo mapping of a GPCR interactome using knockin mice
Abstract
With over 30% of current medications targeting this family of proteins, G-protein-coupled receptors (GPCRs) remain invaluable therapeutic targets. However, due to their unique physicochemical properties, their low abundance, and the lack of highly specific antibodies, GPCRs are still challenging to study in vivo. To overcome these limitations, we combined here transgenic mouse models and proteomic analyses in order to resolve the interactome of the δ-opioid receptor (DOPr) in its native in vivo environment. Given its analgesic properties and milder undesired effects than most clinically prescribed opioids, DOPr is a promising alternative therapeutic target for chronic pain management. However, the molecular and cellular mechanisms regulating its signaling and trafficking remain poorly characterized. We thus performed liquid chromatography-tandem mass spectrometry (LC-MS/MS) analyses on brain homogenates of our newly generated knockin mouse expressing a FLAG-tagged version of DOPr and revealed several endogenous DOPr interactors involved in protein folding, trafficking, and signal transduction. The interactions with a few identified partners such as VPS41, ARF6, Rabaptin-5, and Rab10 were validated. We report an approach to characterize in vivo interacting proteins of GPCRs, the largest family of membrane receptors with crucial implications in virtually all physiological systems.
Keywords: G-protein–coupled receptors; GPCR interactome; mass spectrometry; mouse model; δ-opioid receptor.
Conflict of interest statement
The authors declare no competing interest.
Figures
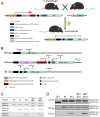
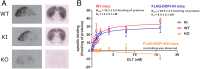
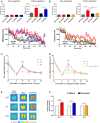
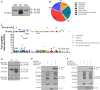
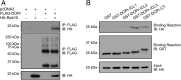
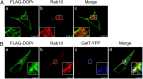
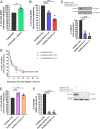
Similar articles
- Endosomal signaling of delta opioid receptors is an endogenous mechanism and therapeutic target for relief from inflammatory pain.Jimenez-Vargas NN, Gong J, Wisdom MJ, Jensen DD, Latorre R, Hegron A, Teng S, DiCello JJ, Rajasekhar P, Veldhuis NA, Carbone SE, Yu Y, Lopez-Lopez C, Jaramillo-Polanco J, Canals M, Reed DE, Lomax AE, Schmidt BL, Leong KW, Vanner SJ, Halls ML, Bunnett NW, Poole DP.Jimenez-Vargas NN, et al.Proc Natl Acad Sci U S A. 2020 Jun 30;117(26):15281-15292. doi: 10.1073/pnas.2000500117. Epub 2020 Jun 16.Proc Natl Acad Sci U S A. 2020.PMID:32546520Free PMC article.
- CHIP-MYTH: a novel interactive proteomics method for the assessment of agonist-dependent interactions of the human β₂-adrenergic receptor.Kittanakom S, Barrios-Rodiles M, Petschnigg J, Arnoldo A, Wong V, Kotlyar M, Heisler LE, Jurisica I, Wrana JL, Nislow C, Stagljar I.Kittanakom S, et al.Biochem Biophys Res Commun. 2014 Mar 21;445(4):746-56. doi: 10.1016/j.bbrc.2014.02.033. Epub 2014 Feb 19.Biochem Biophys Res Commun. 2014.PMID:24561123
- One-step generation of mice carrying a conditional allele together with an HA-tag insertion for the delta opioid receptor.Su D, Wang M, Ye C, Fang J, Duan Y, Zhang Z, Hua Q, Shi C, Zhang L, Zhang R, Xie X.Su D, et al.Sci Rep. 2017 Mar 16;7:44476. doi: 10.1038/srep44476.Sci Rep. 2017.PMID:28300205Free PMC article.
- Knock-In Mouse Models to Investigate the Functions of Opioid Receptorsin vivo.Degrandmaison J, Rochon-Haché S, Parent JL, Gendron L.Degrandmaison J, et al.Front Cell Neurosci. 2022 Jan 31;16:807549. doi: 10.3389/fncel.2022.807549. eCollection 2022.Front Cell Neurosci. 2022.PMID:35173584Free PMC article.Review.
- G Protein-Coupled Receptor Systems as Crucial Regulators of DNA Damage Response Processes.Leysen H, van Gastel J, Hendrickx JO, Santos-Otte P, Martin B, Maudsley S.Leysen H, et al.Int J Mol Sci. 2018 Sep 26;19(10):2919. doi: 10.3390/ijms19102919.Int J Mol Sci. 2018.PMID:30261591Free PMC article.Review.
Cited by
- Delta Opioid Receptor in Astrocytes Contributes to Neuropathic Cold Pain and Analgesic Tolerance in Female Mice.Reiss D, Maurin H, Audouard E, Martínez-Navarro M, Xue Y, Herault Y, Maldonado R, Cabañero D, Gaveriaux-Ruff C.Reiss D, et al.Front Cell Neurosci. 2021 Sep 16;15:745178. doi: 10.3389/fncel.2021.745178. eCollection 2021.Front Cell Neurosci. 2021.PMID:34602984Free PMC article.
- A Rab10-ACAP1-Arf6 GTPases cascade modulates M4 muscarinic acetylcholine receptor trafficking and signaling.Xu R, Wan M, Shi X, Ma S, Zhang L, Yi P, Zhang R.Xu R, et al.Cell Mol Life Sci. 2023 Mar 14;80(4):87. doi: 10.1007/s00018-023-04722-x.Cell Mol Life Sci. 2023.PMID:36917255Free PMC article.
- A proximity labeling method for protein-protein interactions on cell membrane.Li Q, Xie Y, Rice R, Maverakis E, Lebrilla CB.Li Q, et al.Chem Sci. 2022 Apr 30;13(20):6028-6038. doi: 10.1039/d1sc06898a. eCollection 2022 May 25.Chem Sci. 2022.PMID:35685794Free PMC article.
- Genome-wide association study and functional characterization identifies candidate genes for insulin-stimulated glucose uptake.Williamson A, Norris DM, Yin X, Broadaway KA, Moxley AH, Vadlamudi S, Wilson EP, Jackson AU, Ahuja V, Andersen MK, Arzumanyan Z, Bonnycastle LL, Bornstein SR, Bretschneider MP, Buchanan TA, Chang YC, Chuang LM, Chung RH, Clausen TD, Damm P, Delgado GE, de Mello VD, Dupuis J, Dwivedi OP, Erdos MR, Fernandes Silva L, Frayling TM, Gieger C, Goodarzi MO, Guo X, Gustafsson S, Hakaste L, Hammar U, Hatem G, Herrmann S, Højlund K, Horn K, Hsueh WA, Hung YJ, Hwu CM, Jonsson A, Kårhus LL, Kleber ME, Kovacs P, Lakka TA, Lauzon M, Lee IT, Lindgren CM, Lindström J, Linneberg A, Liu CT, Luan J, Aly DM, Mathiesen E, Moissl AP, Morris AP, Narisu N, Perakakis N, Peters A, Prasad RB, Rodionov RN, Roll K, Rundsten CF, Sarnowski C, Savonen K, Scholz M, Sharma S, Stinson SE, Suleman S, Tan J, Taylor KD, Uusitupa M, Vistisen D, Witte DR, Walther R, Wu P, Xiang AH, Zethelius B; Meta-Analysis of Glucose and Insulin-related Traits Consortium (MAGIC); Ahlqvist E, Bergman RN, Chen YI, Collins FS, Fall T, Florez JC, Fritsche A, Grallert H, Groop L, Hansen T, Koistinen HA, Komulainen P, Laakso M, Lind L, Loeffler M, März W, Meigs JB, Raffel LJ, Rauramaa R, Rotter JI, Schwarz PEH, Stumvoll M, Sundström J, Tönj…See abstract for full author list ➔Williamson A, et al.Nat Genet. 2023 Jun;55(6):973-983. doi: 10.1038/s41588-023-01408-9. Epub 2023 Jun 8.Nat Genet. 2023.PMID:37291194Free PMC article.
- Recent advances in basic science methodology to evaluate opioid safety profiles and to understand opioid activities.Ehrlich AT, Darcq E.Ehrlich AT, et al.Fac Rev. 2021 Feb 19;10:15. doi: 10.12703/r/10-15. eCollection 2021.Fac Rev. 2021.PMID:33718932Free PMC article.Review.
References
- Abdallah K., Gendron L., The delta opioid receptor in pain control. Handb. Exp. Pharmacol. 247, 147–177 (2018). - PubMed
- Gallantine E. L., Meert T. F., A comparison of the antinociceptive and adverse effects of the mu-opioid agonist morphine and the delta-opioid agonist SNC80. Basic Clin. Pharmacol. Toxicol. 97, 39–51 (2005). - PubMed
Publication types
MeSH terms
Substances
Related information
Grants and funding
LinkOut - more resources
Full Text Sources
Other Literature Sources
Molecular Biology Databases