Coordinated electrical activity in the olfactory bulb gates the oscillatory entrainment of entorhinal networks in neonatal mice
- PMID:30703080
- PMCID: PMC6354964
- DOI: 10.1371/journal.pbio.2006994
Coordinated electrical activity in the olfactory bulb gates the oscillatory entrainment of entorhinal networks in neonatal mice
Abstract
Although the developmental principles of sensory and cognitive processing have been extensively investigated, their synergy has been largely neglected. During early life, most sensory systems are still largely immature. As a notable exception, the olfactory system is functional at birth, controlling mother-offspring interactions and neonatal survival. Here, we elucidate the structural and functional principles underlying the communication between olfactory bulb (OB) and lateral entorhinal cortex (LEC)-the gatekeeper of limbic circuitry-during neonatal development. Combining optogenetics, pharmacology, and electrophysiology in vivo with axonal tracing, we show that mitral cell-dependent discontinuous theta bursts in OB drive network oscillations and time the firing in LEC of anesthetized mice via axonal projections confined to upper cortical layers. Acute pharmacological silencing of OB activity diminishes entorhinal oscillations, whereas odor exposure boosts OB-entorhinal coupling at fast frequencies. Chronic impairment of olfactory sensory neurons disrupts OB-entorhinal activity. Thus, OB activity shapes the maturation of entorhinal circuits.
Conflict of interest statement
The authors have declared that no competing interests exist.
Figures
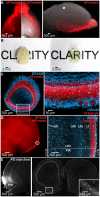
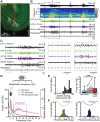
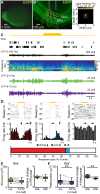
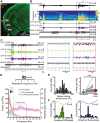
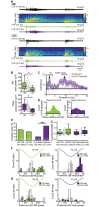
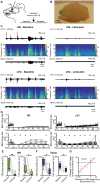
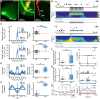
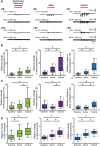
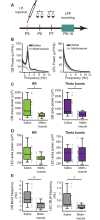
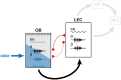
Similar articles
- Olfactory bulb activity shapes the development of entorhinal-hippocampal coupling and associated cognitive abilities.Chen YN, Kostka JK, Bitzenhofer SH, Hanganu-Opatz IL.Chen YN, et al.Curr Biol. 2023 Oct 23;33(20):4353-4366.e5. doi: 10.1016/j.cub.2023.08.072. Epub 2023 Sep 19.Curr Biol. 2023.PMID:37729915Free PMC article.
- Bursting mitral cells time the oscillatory coupling between olfactory bulb and entorhinal networks in neonatal mice.Kostka JK, Gretenkord S, Spehr M, Hanganu-Opatz IL.Kostka JK, et al.J Physiol. 2020 Dec;598(24):5753-5769. doi: 10.1113/JP280131. Epub 2020 Oct 4.J Physiol. 2020.PMID:32926437
- Olfactory-driven beta band entrainment of limbic circuitry during neonatal development.Kostka JK, Hanganu-Opatz IL.Kostka JK, et al.J Physiol. 2023 Aug;601(16):3605-3630. doi: 10.1113/JP284401. Epub 2023 Jul 11.J Physiol. 2023.PMID:37434507
- The olfactory bulb: coding and processing of odor molecule information.Mori K, Nagao H, Yoshihara Y.Mori K, et al.Science. 1999 Oct 22;286(5440):711-5. doi: 10.1126/science.286.5440.711.Science. 1999.PMID:10531048Review.
- Olfactory Circuitry and Behavioral Decisions.Mori K, Sakano H.Mori K, et al.Annu Rev Physiol. 2021 Feb 10;83:231-256. doi: 10.1146/annurev-physiol-031820-092824. Epub 2020 Nov 23.Annu Rev Physiol. 2021.PMID:33228453Review.
Cited by
- How the sense of smell influences cognition throughout life.Kostka JK, Bitzenhofer SH.Kostka JK, et al.Neuroforum. 2022 Aug 26;28(3):177-185. doi: 10.1515/nf-2022-0007. Epub 2022 Jun 6.Neuroforum. 2022.PMID:36067120Free PMC article.Review.
- Olfactory bulb activity shapes the development of entorhinal-hippocampal coupling and associated cognitive abilities.Chen YN, Kostka JK, Bitzenhofer SH, Hanganu-Opatz IL.Chen YN, et al.Curr Biol. 2023 Oct 23;33(20):4353-4366.e5. doi: 10.1016/j.cub.2023.08.072. Epub 2023 Sep 19.Curr Biol. 2023.PMID:37729915Free PMC article.
- Investigating Olfactory Sensory Neurons Facilitation For Aerobic Exercise-induced Spatial Memory Improvement.Zeynali F, Raoufy MR, Gharakhanlou R.Zeynali F, et al.Basic Clin Neurosci. 2024 May-Jun;15(3):355-366. doi: 10.32598/bcn.2022.4029.1. Epub 2024 May 1.Basic Clin Neurosci. 2024.PMID:39403352Free PMC article.
- Stimulus-Induced Theta-Band LFP Oscillations Format Neuronal Representations of Social Chemosignals in the Mouse Accessory Olfactory Bulb.Cohen O, Kahan A, Steinberg I, Malinowski ST, Rokni D, Spehr M, Ben-Shaul Y.Cohen O, et al.J Neurosci. 2023 Dec 13;43(50):8700-8722. doi: 10.1523/JNEUROSCI.1055-23.2023.J Neurosci. 2023.PMID:37903594Free PMC article.
- Generation and propagation of bursts of activity in the developing basal ganglia.Klavinskis-Whiting S, Bitzenhofer S, Hanganu-Opatz I, Ellender T.Klavinskis-Whiting S, et al.Cereb Cortex. 2023 Oct 9;33(20):10595-10613. doi: 10.1093/cercor/bhad307.Cereb Cortex. 2023.PMID:37615347Free PMC article.
References
Publication types
MeSH terms
Related information
LinkOut - more resources
Full Text Sources
Molecular Biology Databases
Research Materials
Miscellaneous