Pluripotent stem cells as a source of osteoblasts for bone tissue regeneration
- PMID:29456164
- PMCID: PMC6077105
- DOI: 10.1016/j.biomaterials.2018.02.009
Pluripotent stem cells as a source of osteoblasts for bone tissue regeneration
Abstract
Appropriate and abundant sources of bone-forming osteoblasts are essential for bone tissue engineering. Pluripotent stem cells can self-renew and thereby offer a potentially unlimited supply of osteoblasts, a significant advantage over other cell sources. We generated mouse embryonic stem cells (ESCs) and induced pluripotent stem cells (iPSCs) from transgenic mice expressing rat 2.3 kb type I collagen promoter-driven green fluorescent protein (Col2.3GFP), a reporter of the osteoblast lineage. We demonstrated that Col2.3GFP ESCs and iPSCs can be successfully differentiated to osteoblast lineage cells that express Col2.3GFP in vitro. We harvested GFP+ osteoblasts differentiated from ESCs. Genome wide gene expression profiles validated that ESC- and iPSC-derived osteoblasts resemble calvarial osteoblasts, and that Col2.3GFP expression serves as a marker for mature osteoblasts. Our results confirm the cell identity of ESC- and iPSC-derived osteoblasts and highlight the potential of pluripotent stem cells as a source of osteoblasts for regenerative medicine.
Keywords: Differentiation; Embryonic stem cells; Induced pluripotent stem cells; Osteoblasts; Tissue engineering.
Copyright © 2018 Elsevier Ltd. All rights reserved.
Figures
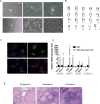
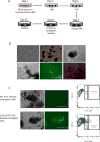
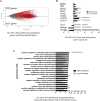
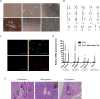
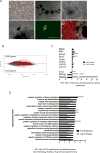
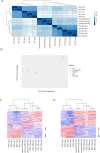
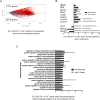
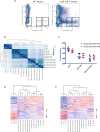
Similar articles
- Direct Reprogramming of Mouse Fibroblasts into Functional Osteoblasts.Zhu H, Swami S, Yang P, Shapiro F, Wu JY.Zhu H, et al.J Bone Miner Res. 2020 Apr;35(4):698-713. doi: 10.1002/jbmr.3929. Epub 2019 Dec 30.J Bone Miner Res. 2020.PMID:31793059Free PMC article.
- A Site-Specific Integrated Col2.3GFP Reporter Identifies Osteoblasts Within Mineralized Tissue Formed In Vivo by Human Embryonic Stem Cells.Xin X, Jiang X, Wang L, Stover ML, Zhan S, Huang J, Goldberg AJ, Liu Y, Kuhn L, Reichenberger EJ, Rowe DW, Lichtler AC.Xin X, et al.Stem Cells Transl Med. 2014 Oct;3(10):1125-37. doi: 10.5966/sctm.2013-0128. Epub 2014 Aug 13.Stem Cells Transl Med. 2014.PMID:25122686Free PMC article.
- Induction of Osteoblasts by Direct Reprogramming of Mouse Fibroblasts.Zhu H, Wu JY.Zhu H, et al.Methods Mol Biol. 2020;2155:201-212. doi: 10.1007/978-1-0716-0655-1_17.Methods Mol Biol. 2020.PMID:32474879
- Induced Pluripotent Stem Cells as a new Strategy for Osteogenesis and Bone Regeneration.Lou X.Lou X.Stem Cell Rev Rep. 2015 Aug;11(4):645-51. doi: 10.1007/s12015-015-9594-8.Stem Cell Rev Rep. 2015.PMID:26022504Review.
- Reverse engineering human neurodegenerative disease using pluripotent stem cell technology.Liu Y, Deng W.Liu Y, et al.Brain Res. 2016 May 1;1638(Pt A):30-41. doi: 10.1016/j.brainres.2015.09.023. Epub 2015 Sep 28.Brain Res. 2016.PMID:26423934Free PMC article.Review.
Cited by
- Direct Reprogramming of Mouse Fibroblasts into Functional Osteoblasts.Zhu H, Swami S, Yang P, Shapiro F, Wu JY.Zhu H, et al.J Bone Miner Res. 2020 Apr;35(4):698-713. doi: 10.1002/jbmr.3929. Epub 2019 Dec 30.J Bone Miner Res. 2020.PMID:31793059Free PMC article.
- Gene-repaired iPS cells as novel approach for patient withosteogenesis imperfecta.Fus-Kujawa A, Mendrek B, Bajdak-Rusinek K, Diak N, Strzelec K, Gutmajster E, Janelt K, Kowalczuk A, Trybus A, Rozwadowska P, Wojakowski W, Gawron K, Sieroń AL.Fus-Kujawa A, et al.Front Bioeng Biotechnol. 2023 Jun 30;11:1205122. doi: 10.3389/fbioe.2023.1205122. eCollection 2023.Front Bioeng Biotechnol. 2023.PMID:37456734Free PMC article.
- The role of vesicle trafficking genes in osteoblast differentiation and function.Zhu H, Su Y, Wang J, Wu JY.Zhu H, et al.Sci Rep. 2023 Sep 26;13(1):16079. doi: 10.1038/s41598-023-43116-8.Sci Rep. 2023.PMID:37752218Free PMC article.
- Bone Repair and Regenerative Biomaterials: Towards Recapitulating the Microenvironment.Aslankoohi N, Mondal D, Rizkalla AS, Mequanint K.Aslankoohi N, et al.Polymers (Basel). 2019 Sep 2;11(9):1437. doi: 10.3390/polym11091437.Polymers (Basel). 2019.PMID:31480693Free PMC article.Review.
- Adipose-derived mesenchymal stem cells (MSCs) are a superior cell source for bone tissue engineering.Gou Y, Huang Y, Luo W, Li Y, Zhao P, Zhong J, Dong X, Guo M, Li A, Hao A, Zhao G, Wang Y, Zhu Y, Zhang H, Shi Y, Wagstaff W, Luu HH, Shi LL, Reid RR, He TC, Fan J.Gou Y, et al.Bioact Mater. 2023 Dec 14;34:51-63. doi: 10.1016/j.bioactmat.2023.12.003. eCollection 2024 Apr.Bioact Mater. 2023.PMID:38186960Free PMC article.
References
- Pittenger MF, Mackay AM, Beck SC, Jaiswal RK, Douglas R, Mosca JD, Moorman MA, Simonetti DW, Craig S, Marshak DR. Multilineage potential of adult human mesenchymal stem cells. Science. 1999;284(5411):143–7. - PubMed
- Siddappa R, Licht R, van Blitterswijk C, de Boer J. Donor variation and loss of multipotency during in vitro expansion of human mesenchymal stem cells for bone tissue engineering. J Orthop Res. 2007;25(8):1029–41. - PubMed
Publication types
MeSH terms
Substances
Related information
Grants and funding
LinkOut - more resources
Full Text Sources
Other Literature Sources
Molecular Biology Databases