The state of play in higher eukaryote gene annotation
- PMID:27773922
- PMCID: PMC5876476
- DOI: 10.1038/nrg.2016.119
The state of play in higher eukaryote gene annotation
Abstract
A genome sequence is worthless if it cannot be deciphered; therefore, efforts to describe - or 'annotate' - genes began as soon as DNA sequences became available. Whereas early work focused on individual protein-coding genes, the modern genomic ocean is a complex maelstrom of alternative splicing, non-coding transcription and pseudogenes. Scientists - from clinicians to evolutionary biologists - need to navigate these waters, and this has led to the design of high-throughput, computationally driven annotation projects. The catalogues that are being produced are key resources for genome exploration, especially as they become integrated with expression, epigenomic and variation data sets. Their creation, however, remains challenging.
Figures
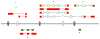
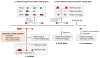
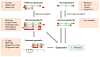
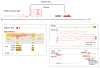
Similar articles
- Roadmap for annotating transposable elements in eukaryote genomes.Permal E, Flutre T, Quesneville H.Permal E, et al.Methods Mol Biol. 2012;859:53-68. doi: 10.1007/978-1-61779-603-6_3.Methods Mol Biol. 2012.PMID:22367865
- GENCODE Pseudogenes.Sisu C.Sisu C.Methods Mol Biol. 2021;2324:67-82. doi: 10.1007/978-1-0716-1503-4_5.Methods Mol Biol. 2021.PMID:34165709
- Computational Methods for Pseudogene Annotation Based on Sequence Homology.Harrison PM.Harrison PM.Methods Mol Biol. 2021;2324:35-48. doi: 10.1007/978-1-0716-1503-4_3.Methods Mol Biol. 2021.PMID:34165707Review.
- Segway 2.0: Gaussian mixture models and minibatch training.Chan RCW, Libbrecht MW, Roberts EG, Bilmes JA, Noble WS, Hoffman MM.Chan RCW, et al.Bioinformatics. 2018 Feb 15;34(4):669-671. doi: 10.1093/bioinformatics/btx603.Bioinformatics. 2018.PMID:29028889Free PMC article.
- A beginner's guide to eukaryotic genome annotation.Yandell M, Ence D.Yandell M, et al.Nat Rev Genet. 2012 Apr 18;13(5):329-42. doi: 10.1038/nrg3174.Nat Rev Genet. 2012.PMID:22510764Review.
Cited by
- Recognition of the polycistronic nature of human genes is critical to understanding the genotype-phenotype relationship.Brunet MA, Levesque SA, Hunting DJ, Cohen AA, Roucou X.Brunet MA, et al.Genome Res. 2018 May;28(5):609-624. doi: 10.1101/gr.230938.117. Epub 2018 Apr 6.Genome Res. 2018.PMID:29626081Free PMC article.Review.
- De novo assembly and annotation of the Patagonian toothfish (Dissostichus eleginoides) genome.Ryder D, Stone D, Minardi D, Riley A, Avant J, Cross L, Soeffker M, Davidson D, Newman A, Thomson P, Darby C, van Aerle R.Ryder D, et al.BMC Genomics. 2024 Mar 4;25(1):233. doi: 10.1186/s12864-024-10141-4.BMC Genomics. 2024.PMID:38438840Free PMC article.
- ToxCodAn-Genome: an automated pipeline for toxin-gene annotation in genome assembly of venomous lineages.Nachtigall PG, Durham AM, Rokyta DR, Junqueira-de-Azevedo ILM.Nachtigall PG, et al.Gigascience. 2024 Jan 2;13:giad116. doi: 10.1093/gigascience/giad116.Gigascience. 2024.PMID:38241143Free PMC article.
- TEx-MST: tissue expression profiles of MANE select transcripts.Tung KF, Lin WC.Tung KF, et al.Database (Oxford). 2022 Sep 28;2022:baac089. doi: 10.1093/database/baac089.Database (Oxford). 2022.PMID:36170113Free PMC article.
- Discovery of high-confidence human protein-coding genes and exons by whole-genome PhyloCSF helps elucidate 118 GWAS loci.Mudge JM, Jungreis I, Hunt T, Gonzalez JM, Wright JC, Kay M, Davidson C, Fitzgerald S, Seal R, Tweedie S, He L, Waterhouse RM, Li Y, Bruford E, Choudhary JS, Frankish A, Kellis M.Mudge JM, et al.Genome Res. 2019 Dec;29(12):2073-2087. doi: 10.1101/gr.246462.118. Epub 2019 Sep 19.Genome Res. 2019.PMID:31537640Free PMC article.
References
- Kim VN, Han J, Siomi MC. Biogenesis of small RNAs in animals. Nat Rev Mol Cell Biol. 2009;10:126–39. - PubMed
Publication types
MeSH terms
Related information
Grants and funding
LinkOut - more resources
Full Text Sources
Other Literature Sources