Molecular Pharmacology of δ-Opioid Receptors
- PMID:27343248
- PMCID: PMC4931872
- DOI: 10.1124/pr.114.008979
Molecular Pharmacology of δ-Opioid Receptors
Abstract
Opioids are among the most effective analgesics available and are the first choice in the treatment of acute severe pain. However, partial efficacy, a tendency to produce tolerance, and a host of ill-tolerated side effects make clinically available opioids less effective in the management of chronic pain syndromes. Given that most therapeutic opioids produce their actions via µ-opioid receptors (MOPrs), other targets are constantly being explored, among which δ-opioid receptors (DOPrs) are being increasingly considered as promising alternatives. This review addresses DOPrs from the perspective of cellular and molecular determinants of their pharmacological diversity. Thus, DOPr ligands are examined in terms of structural and functional variety, DOPrs' capacity to engage a multiplicity of canonical and noncanonical G protein-dependent responses is surveyed, and evidence supporting ligand-specific signaling and regulation is analyzed. Pharmacological DOPr subtypes are examined in light of the ability of DOPr to organize into multimeric arrays and to adopt multiple active conformations as well as differences in ligand kinetics. Current knowledge on DOPr targeting to the membrane is examined as a means of understanding how these receptors are especially active in chronic pain management. Insight into cellular and molecular mechanisms of pharmacological diversity should guide the rational design of more effective, longer-lasting, and better-tolerated opioid analgesics for chronic pain management.
Copyright © 2016 by The American Society for Pharmacology and Experimental Therapeutics.
Figures
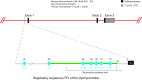
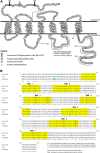
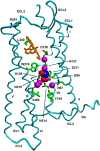
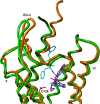
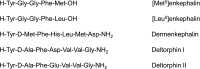
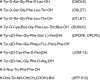
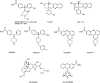
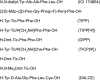
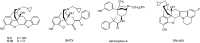
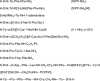
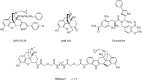
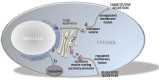
Similar articles
- Gadolinium Magnetic Resonance Imaging.Ibrahim MA, Hazhirkarzar B, Dublin AB.Ibrahim MA, et al.2023 Jul 3. In: StatPearls [Internet]. Treasure Island (FL): StatPearls Publishing; 2025 Jan–.2023 Jul 3. In: StatPearls [Internet]. Treasure Island (FL): StatPearls Publishing; 2025 Jan–.PMID:29494094Free Books & Documents.
- Exploring the impact of housing insecurity on the health and well-being of children and young people: a systematic review.Hock E, Blank L, Fairbrother H, Clowes M, Cuevas DC, Booth A, Goyder E.Hock E, et al.Public Health Res (Southampt). 2023 Dec;11(13):1-71. doi: 10.3310/TWWL4501.Public Health Res (Southampt). 2023.PMID:39789922
- Exploring conceptual and theoretical frameworks for nurse practitioner education: a scoping review protocol.Wilson R, Godfrey CM, Sears K, Medves J, Ross-White A, Lambert N.Wilson R, et al.JBI Database System Rev Implement Rep. 2015 Oct;13(10):146-55. doi: 10.11124/jbisrir-2015-2150.JBI Database System Rev Implement Rep. 2015.PMID:26571290
- Pain, Motivation, Migraine, and the Microbiome: New Frontiers for Opioid Systems and Disease.Parker KE, Sugiarto E, Taylor AMW, Pradhan AA, Al-Hasani R.Parker KE, et al.Mol Pharmacol. 2020 Oct;98(4):433-444. doi: 10.1124/mol.120.119438. Epub 2020 Jul 27.Mol Pharmacol. 2020.PMID:32958571Free PMC article.Review.
- Effectiveness of interventions for improving educational outcomes for people with disabilities in low- and middle-income countries: A systematic review.Hunt X, Saran A, White H, Kuper H.Hunt X, et al.Campbell Syst Rev. 2025 Feb 6;21(1):e70016. doi: 10.1002/cl2.70016. eCollection 2025 Mar.Campbell Syst Rev. 2025.PMID:39917627Free PMC article.Review.
Cited by
- Neuropeptide and cytokine regulation of pain in the context of substance use disorders.Delery EC, Edwards S.Delery EC, et al.Neuropharmacology. 2020 Sep 1;174:108153. doi: 10.1016/j.neuropharm.2020.108153. Epub 2020 May 26.Neuropharmacology. 2020.PMID:32470337Free PMC article.Review.
- LP1 and LP2: Dual-Target MOPr/DOPr Ligands as Drug Candidates for Persistent Pain Relief.Pasquinucci L, Parenti C, Georgoussi Z, Reina L, Tomarchio E, Turnaturi R.Pasquinucci L, et al.Molecules. 2021 Jul 8;26(14):4168. doi: 10.3390/molecules26144168.Molecules. 2021.PMID:34299443Free PMC article.Review.
- A PTEN-Regulated Checkpoint Controls Surface Delivery of δ Opioid Receptors.Shiwarski DJ, Tipton A, Giraldo MD, Schmidt BF, Gold MS, Pradhan AA, Puthenveedu MA.Shiwarski DJ, et al.J Neurosci. 2017 Apr 5;37(14):3741-3752. doi: 10.1523/JNEUROSCI.2923-16.2017. Epub 2017 Mar 6.J Neurosci. 2017.PMID:28264976Free PMC article.
- Dual RXR motifs regulate nerve growth factor-mediated intracellular retention of the delta opioid receptor.Shiwarski DJ, Crilly SE, Dates A, Puthenveedu MA.Shiwarski DJ, et al.Mol Biol Cell. 2019 Mar 1;30(5):680-690. doi: 10.1091/mbc.E18-05-0292. Epub 2019 Jan 2.Mol Biol Cell. 2019.PMID:30601694Free PMC article.
- In vivo mapping of a GPCR interactome using knockin mice.Degrandmaison J, Abdallah K, Blais V, Génier S, Lalumière MP, Bergeron F, Cahill CM, Boulter J, Lavoie CL, Parent JL, Gendron L.Degrandmaison J, et al.Proc Natl Acad Sci U S A. 2020 Jun 9;117(23):13105-13116. doi: 10.1073/pnas.1917906117. Epub 2020 May 26.Proc Natl Acad Sci U S A. 2020.PMID:32457152Free PMC article.
References
- Abdelhamid EE, Sultana M, Portoghese PS, Takemori AE. (1991) Selective blockage of delta opioid receptors prevents the development of morphine tolerance and dependence in mice. J Pharmacol Exp Ther 258:299–303. - PubMed
- Abu-Helo A, Simonin F. (2010) Identification and biological significance of G protein-coupled receptor associated sorting proteins (GASPs). Pharmacol Ther 126:244–250. - PubMed
- Ahuja S, Smith SO. (2009) Multiple switches in G protein-coupled receptor activation. Trends Pharmacol Sci 30:494–502. - PubMed
- Allouche S, Hasbi A, Ferey V, Sola B, Jauzac P, Polastron J. (2000) Pharmacological delta1- and delta2-opioid receptor subtypes in the human neuroblastoma cell line SK-N-BE: no evidence for distinct molecular entities. Biochem Pharmacol 59:915–925. - PubMed