Lrp4 in astrocytes modulates glutamatergic transmission
- PMID:27294513
- PMCID: PMC4961622
- DOI: 10.1038/nn.4326
Lrp4 in astrocytes modulates glutamatergic transmission
Abstract
Neurotransmission requires precise control of neurotransmitter release from axon terminals. This process is regulated by glial cells; however, the underlying mechanisms are not fully understood. We found that glutamate release in the brain was impaired in mice lacking low-density lipoprotein receptor-related protein 4 (Lrp4), a protein that is critical for neuromuscular junction formation. Electrophysiological studies revealed compromised release probability in astrocyte-specific Lrp4 knockout mice. Lrp4 mutant astrocytes suppressed glutamatergic transmission by enhancing the release of ATP, whose level was elevated in the hippocampus of Lrp4 mutant mice. Consequently, the mutant mice were impaired in locomotor activity and spatial memory and were resistant to seizure induction. These impairments could be ameliorated by blocking the adenosine A1 receptor. The results reveal a critical role for Lrp4, in response to agrin, in modulating astrocytic ATP release and synaptic transmission. Our findings provide insight into the interaction between neurons and astrocytes for synaptic homeostasis and/or plasticity.
Figures
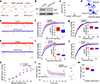
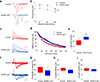
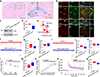
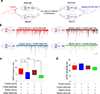
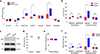
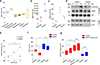
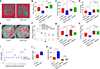
Similar articles
- LRP4 serves as a coreceptor of agrin.Zhang B, Luo S, Wang Q, Suzuki T, Xiong WC, Mei L.Zhang B, et al.Neuron. 2008 Oct 23;60(2):285-97. doi: 10.1016/j.neuron.2008.10.006.Neuron. 2008.PMID:18957220Free PMC article.
- Astrocytic Lrp4 (Low-Density Lipoprotein Receptor-Related Protein 4) Contributes to Ischemia-Induced Brain Injury by Regulating ATP Release and Adenosine-A2AR (Adenosine A2A Receptor) Signaling.Ye XC, Hu JX, Li L, Li Q, Tang FL, Lin S, Sun D, Sun XD, Cui GY, Mei L, Xiong WC.Ye XC, et al.Stroke. 2018 Jan;49(1):165-174. doi: 10.1161/STROKEAHA.117.018115. Epub 2017 Dec 6.Stroke. 2018.PMID:29212737Free PMC article.
- The role of agrin, Lrp4 and MuSK during dendritic arborization and synaptogenesis in cultured embryonic CNS neurons.Handara G, Hetsch FJA, Jüttner R, Schick A, Haupt C, Rathjen FG, Kröger S.Handara G, et al.Dev Biol. 2019 Jan 1;445(1):54-67. doi: 10.1016/j.ydbio.2018.10.017. Epub 2018 Oct 29.Dev Biol. 2019.PMID:30385274
- New dogs in the dogma: Lrp4 and Tid1 in neuromuscular synapse formation.Song Y, Balice-Gordon R.Song Y, et al.Neuron. 2008 Nov 26;60(4):526-8. doi: 10.1016/j.neuron.2008.11.003.Neuron. 2008.PMID:19038209Review.
- New roles for astrocytes: regulation of synaptic transmission.Newman EA.Newman EA.Trends Neurosci. 2003 Oct;26(10):536-42. doi: 10.1016/S0166-2236(03)00237-6.Trends Neurosci. 2003.PMID:14522146Review.
Cited by
- Transmembrane protein 108 inhibits the proliferation and myelination of oligodendrocyte lineage cells in the corpus callosum.Wu Y, Zhong Y, Liao X, Miao X, Yu J, Lai X, Zhang Y, Ma C, Pan H, Wang S.Wu Y, et al.Mol Brain. 2022 Apr 11;15(1):33. doi: 10.1186/s13041-022-00918-7.Mol Brain. 2022.PMID:35410424Free PMC article.
- Amyloid β Clearance Is Disrupted by Depletion of Low-Density Lipoprotein Receptor-Related Protein 4 (LRP4) in Astrocytes.Cavieres-Lepe J, Stuardo N.Cavieres-Lepe J, et al.J Neurosci. 2021 Apr 28;41(17):3749-3751. doi: 10.1523/JNEUROSCI.2352-20.2021.J Neurosci. 2021.PMID:33910985Free PMC article.No abstract available.
- Ablation of Lrp4 in Schwann Cells Promotes Peripheral Nerve Regeneration in Mice.Hui TK, Lai XS, Dong X, Jing H, Liu Z, Fei E, Chen WB, Wang S, Ren D, Zou S, Wu HT, Pan BX.Hui TK, et al.Biology (Basel). 2021 May 21;10(6):452. doi: 10.3390/biology10060452.Biology (Basel). 2021.PMID:34063992Free PMC article.
- Astrocytic lactate dehydrogenase A regulates neuronal excitability and depressive-like behaviors through lactate homeostasis in mice.Yao S, Xu MD, Wang Y, Zhao ST, Wang J, Chen GF, Chen WB, Liu J, Huang GB, Sun WJ, Zhang YY, Hou HL, Li L, Sun XD.Yao S, et al.Nat Commun. 2023 Feb 9;14(1):729. doi: 10.1038/s41467-023-36209-5.Nat Commun. 2023.PMID:36759610Free PMC article.
- Transient Astrocytic Gq Signaling Underlies Remote Memory Enhancement.Iwai Y, Ozawa K, Yahagi K, Mishima T, Akther S, Vo CT, Lee AB, Tanaka M, Itohara S, Hirase H.Iwai Y, et al.Front Neural Circuits. 2021 Mar 22;15:658343. doi: 10.3389/fncir.2021.658343. eCollection 2021.Front Neural Circuits. 2021.PMID:33828463Free PMC article.
References
References for main text
- Haydon PG. GLIA: listening and talking to the synapse. Nature reviews. Neuroscience. 2001;2:185–193. - PubMed
- Perea G, Navarrete M, Araque A. Tripartite synapses: astrocytes process and control synaptic information. Trends in neurosciences. 2009;32:421–431. - PubMed
- Azevedo FA, et al. Equal numbers of neuronal and nonneuronal cells make the human brain an isometrically scaled-up primate brain. The Journal of comparative neurology. 2009;513:532–541. - PubMed
Methods-only references
- Zhuo L, et al. hGFAP-cre transgenic mice for manipulation of glial and neuronal function in vivo. Genesis (New York, N.Y. : 2000) 2001;31:85–94. - PubMed
- Tsien JZ, et al. Subregion- and cell type-restricted gene knockout in mouse brain. Cell. 1996;87:1317–1326. - PubMed
- Casper KB, Jones K, McCarthy KD. Characterization of astrocyte-specific conditional knockouts. Genesis (New York, N.Y. : 2000) 2007;45:292–299. - PubMed
MeSH terms
Substances
Related information
Grants and funding
LinkOut - more resources
Full Text Sources
Other Literature Sources
Molecular Biology Databases
Miscellaneous