Global Rebalancing of Cellular Resources by Pleiotropic Point Mutations Illustrates a Multi-scale Mechanism of Adaptive Evolution
- PMID:27135538
- PMCID: PMC4853925
- DOI: 10.1016/j.cels.2016.04.003
Global Rebalancing of Cellular Resources by Pleiotropic Point Mutations Illustrates a Multi-scale Mechanism of Adaptive Evolution
Abstract
Pleiotropic regulatory mutations affect diverse cellular processes, posing a challenge to our understanding of genotype-phenotype relationships across multiple biological scales. Adaptive laboratory evolution (ALE) allows for such mutations to be found and characterized in the context of clear selection pressures. Here, several ALE-selected single-mutation variants in RNA polymerase (RNAP) of Escherichia coli are detailed using an integrated multi-scale experimental and computational approach. While these mutations increase cellular growth rates in steady environments, they reduce tolerance to stress and environmental fluctuations. We detail structural changes in the RNAP that rewire the transcriptional machinery to rebalance proteome and energy allocation toward growth and away from several hedging and stress functions. We find that while these mutations occur in diverse locations in the RNAP, they share a common adaptive mechanism. In turn, these findings highlight the resource allocation trade-offs organisms face and suggest how the structure of the regulatory network enhances evolvability.
Copyright © 2016 Elsevier Inc. All rights reserved.
Figures
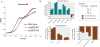
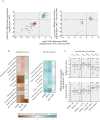
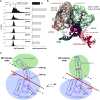
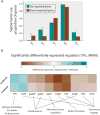
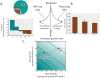
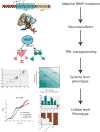
Similar articles
- The hallmarks of a tradeoff in transcriptomes that balances stress and growth functions.Dalldorf C, Rychel K, Szubin R, Hefner Y, Patel A, Zielinski DC, Palsson BO.Dalldorf C, et al.mSystems. 2024 Jul 23;9(7):e0030524. doi: 10.1128/msystems.00305-24. Epub 2024 Jun 3.mSystems. 2024.PMID:38829048Free PMC article.
- Causal mutations from adaptive laboratory evolution are outlined by multiple scales of genome annotations and condition-specificity.Phaneuf PV, Yurkovich JT, Heckmann D, Wu M, Sandberg TE, King ZA, Tan J, Palsson BO, Feist AM.Phaneuf PV, et al.BMC Genomics. 2020 Jul 25;21(1):514. doi: 10.1186/s12864-020-06920-4.BMC Genomics. 2020.PMID:32711472Free PMC article.
- Genotype-by-environment interactions due to antibiotic resistance and adaptation in Escherichia coli.Hall AR.Hall AR.J Evol Biol. 2013 Aug;26(8):1655-64. doi: 10.1111/jeb.12172. Epub 2013 May 23.J Evol Biol. 2013.PMID:23701170
- The Old and New Testaments of gene regulation. Evolution of multi-subunit RNA polymerases and co-evolution of eukaryote complexity with the RNAP II CTD.Burton ZF.Burton ZF.Transcription. 2014;5(3):e28674. doi: 10.4161/trns.28674.Transcription. 2014.PMID:25764332Free PMC article.Review.
- Molecular and cellular bases of adaptation to a changing environment in microorganisms.Bleuven C, Landry CR.Bleuven C, et al.Proc Biol Sci. 2016 Oct 26;283(1841):20161458. doi: 10.1098/rspb.2016.1458.Proc Biol Sci. 2016.PMID:27798299Free PMC article.Review.
Cited by
- Revealing 29 sets of independently modulated genes inStaphylococcus aureus, their regulators, and role in key physiological response.Poudel S, Tsunemoto H, Seif Y, Sastry AV, Szubin R, Xu S, Machado H, Olson CA, Anand A, Pogliano J, Nizet V, Palsson BO.Poudel S, et al.Proc Natl Acad Sci U S A. 2020 Jul 21;117(29):17228-17239. doi: 10.1073/pnas.2008413117. Epub 2020 Jul 2.Proc Natl Acad Sci U S A. 2020.PMID:32616573Free PMC article.
- The Escherichia coli transcriptome mostly consists of independently regulated modules.Sastry AV, Gao Y, Szubin R, Hefner Y, Xu S, Kim D, Choudhary KS, Yang L, King ZA, Palsson BO.Sastry AV, et al.Nat Commun. 2019 Dec 4;10(1):5536. doi: 10.1038/s41467-019-13483-w.Nat Commun. 2019.PMID:31797920Free PMC article.
- Resource allocation accounts for the large variability of rate-yield phenotypes across bacterial strains.Baldazzi V, Ropers D, Gouzé JL, Gedeon T, de Jong H.Baldazzi V, et al.Elife. 2023 May 31;12:e79815. doi: 10.7554/eLife.79815.Elife. 2023.PMID:37255080Free PMC article.
- How adaptive evolution reshapes metabolism to improve fitness: recent advances and future outlook.Long CP, Antoniewicz MR.Long CP, et al.Curr Opin Chem Eng. 2018 Dec;22:209-215. doi: 10.1016/j.coche.2018.11.001. Epub 2018 Nov 26.Curr Opin Chem Eng. 2018.PMID:30613467Free PMC article.
- Reframing gene essentiality in terms of adaptive flexibility.Guzmán GI, Olson CA, Hefner Y, Phaneuf PV, Catoiu E, Crepaldi LB, Micas LG, Palsson BO, Feist AM.Guzmán GI, et al.BMC Syst Biol. 2018 Dec 17;12(1):143. doi: 10.1186/s12918-018-0653-z.BMC Syst Biol. 2018.PMID:30558585Free PMC article.
References
- Bar-Nahum G, Epshtein V, Ruckenstein AE, Rafikov R, Mustaev A, Nudler E. A ratchet mechanism of transcription elongation and its control. Cell. 2005;120:183–193. - PubMed
- Barker MM, Gaal T, Gourse RL. Mechanism of regulation of transcription initiation by ppGpp. II. Models for positive control based on properties of RNAP mutants and competition for RNAP. Journal of molecular biology. 2001;305:689–702. - PubMed
- Cashel M, Hsu LM, Hernandez VJ. Changes in conserved region 3 of Escherichia coli sigma 70 reduce abortive transcription and enhance promoter escape. J Biol Chem. 2003;278:5539–5547. - PubMed
Publication types
MeSH terms
Substances
Related information
Grants and funding
LinkOut - more resources
Full Text Sources
Other Literature Sources
Molecular Biology Databases