Lysosomal Disorders Drive Susceptibility to Tuberculosis by Compromising Macrophage Migration
- PMID:27015311
- PMCID: PMC4819607
- DOI: 10.1016/j.cell.2016.02.034
Lysosomal Disorders Drive Susceptibility to Tuberculosis by Compromising Macrophage Migration
Abstract
A zebrafish genetic screen for determinants of susceptibility to Mycobacterium marinum identified a hypersusceptible mutant deficient in lysosomal cysteine cathepsins that manifests hallmarks of human lysosomal storage diseases. Under homeostatic conditions, mutant macrophages accumulate undigested lysosomal material, which disrupts endocytic recycling and impairs their migration to, and thus engulfment of, dying cells. This causes a buildup of unengulfed cell debris. During mycobacterial infection, macrophages with lysosomal storage cannot migrate toward infected macrophages undergoing apoptosis in the tuberculous granuloma. The unengulfed apoptotic macrophages undergo secondary necrosis, causing granuloma breakdown and increased mycobacterial growth. Macrophage lysosomal storage similarly impairs migration to newly infecting mycobacteria. This phenotype is recapitulated in human smokers, who are at increased risk for tuberculosis. A majority of their alveolar macrophages exhibit lysosomal accumulations of tobacco smoke particulates and do not migrate to Mycobacterium tuberculosis. The incapacitation of highly microbicidal first-responding macrophages may contribute to smokers' susceptibility to tuberculosis.
Copyright © 2016 The Authors. Published by Elsevier Inc. All rights reserved.
Figures
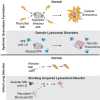
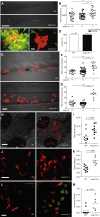
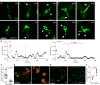
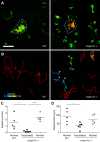
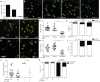
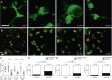
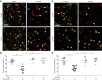
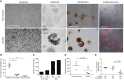
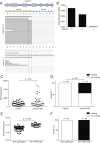
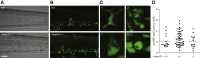
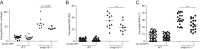
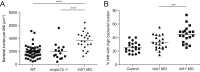
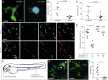
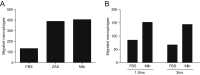
Comment in
- Adding Insult to Injury: Exacerbating TB Risk with Smoking.Glickman MS, Schluger N.Glickman MS, et al.Cell Host Microbe. 2016 Apr 13;19(4):432-3. doi: 10.1016/j.chom.2016.04.001.Cell Host Microbe. 2016.PMID:27078065Free PMC article.
Similar articles
- The role of the granuloma in expansion and dissemination of early tuberculous infection.Davis JM, Ramakrishnan L.Davis JM, et al.Cell. 2009 Jan 9;136(1):37-49. doi: 10.1016/j.cell.2008.11.014.Cell. 2009.PMID:19135887Free PMC article.
- Disruption of Cxcr3 chemotactic signaling alters lysosomal function and renders macrophages more microbicidal.Sommer F, Torraca V, Xie Y, In 't Veld AE, Willemse J, Meijer AH.Sommer F, et al.Cell Rep. 2021 Apr 13;35(2):109000. doi: 10.1016/j.celrep.2021.109000.Cell Rep. 2021.PMID:33852860
- Linking Smokers' Susceptibility to Tuberculosis with Lysosomal Storage Disorders.Meijer AH, Aerts JM.Meijer AH, et al.Dev Cell. 2016 Apr 18;37(2):112-3. doi: 10.1016/j.devcel.2016.04.004.Dev Cell. 2016.PMID:27093080Review.
- The CXCR3-CXCL11 signaling axis mediates macrophage recruitment and dissemination of mycobacterial infection.Torraca V, Cui C, Boland R, Bebelman JP, van der Sar AM, Smit MJ, Siderius M, Spaink HP, Meijer AH.Torraca V, et al.Dis Model Mech. 2015 Mar;8(3):253-69. doi: 10.1242/dmm.017756. Epub 2015 Jan 8.Dis Model Mech. 2015.PMID:25573892Free PMC article.
- Insights into early mycobacterial pathogenesis from the zebrafish.Lesley R, Ramakrishnan L.Lesley R, et al.Curr Opin Microbiol. 2008 Jun;11(3):277-83. doi: 10.1016/j.mib.2008.05.013. Epub 2008 Jun 19.Curr Opin Microbiol. 2008.PMID:18571973Free PMC article.Review.
Cited by
- CD11cHi monocyte-derived macrophages are a major cellular compartment infected by Mycobacterium tuberculosis.Lee J, Boyce S, Powers J, Baer C, Sassetti CM, Behar SM.Lee J, et al.PLoS Pathog. 2020 Jun 16;16(6):e1008621. doi: 10.1371/journal.ppat.1008621. eCollection 2020 Jun.PLoS Pathog. 2020.PMID:32544188Free PMC article.
- mTOR-regulated mitochondrial metabolism limits mycobacterium-induced cytotoxicity.Pagán AJ, Lee LJ, Edwards-Hicks J, Moens CB, Tobin DM, Busch-Nentwich EM, Pearce EL, Ramakrishnan L.Pagán AJ, et al.Cell. 2022 Sep 29;185(20):3720-3738.e13. doi: 10.1016/j.cell.2022.08.018. Epub 2022 Sep 13.Cell. 2022.PMID:36103894Free PMC article.
- Lysosome (Dys)function in Atherosclerosis-A Big Weight on the Shoulders of a Small Organelle.Marques ARA, Ramos C, Machado-Oliveira G, Vieira OV.Marques ARA, et al.Front Cell Dev Biol. 2021 Mar 29;9:658995. doi: 10.3389/fcell.2021.658995. eCollection 2021.Front Cell Dev Biol. 2021.PMID:33855029Free PMC article.Review.
- Bi-allelic variants in HOPS complex subunit VPS41 cause cerebellar ataxia and abnormal membrane trafficking.Sanderson LE, Lanko K, Alsagob M, Almass R, Al-Ahmadi N, Najafi M, Al-Muhaizea MA, Alzaidan H, AlDhalaan H, Perenthaler E, van der Linde HC, Nikoncuk A, Kühn NA, Antony D, Owaidah TM, Raskin S, Vieira LGDR, Mombach R, Ahangari N, Silveira TRD, Ameziane N, Rolfs A, Alharbi A, Sabbagh RM, AlAhmadi K, Alawam B, Ghebeh H, AlHargan A, Albader AA, Binhumaid FS, Goljan E, Monies D, Mustafa OM, Aldosary M, AlBakheet A, Alyounes B, Almutairi F, Al-Odaib A, Aksoy DB, Basak AN, Palvadeau R, Trabzuni D, Rosenfeld JA, Karimiani EG, Meyer BF, Karakas B, Al-Mohanna F, Arold ST, Colak D, Maroofian R, Houlden H, Bertoli-Avella AM, Schmidts M, Barakat TS, van Ham TJ, Kaya N.Sanderson LE, et al.Brain. 2021 Apr 12;144(3):769-780. doi: 10.1093/brain/awaa459.Brain. 2021.PMID:33764426Free PMC article.
- Targeting immunometabolism in host defence against Mycobacterium tuberculosis.Sheedy FJ, Divangahi M.Sheedy FJ, et al.Immunology. 2021 Feb;162(2):145-159. doi: 10.1111/imm.13276. Epub 2020 Oct 28.Immunology. 2021.PMID:33020911Free PMC article.Review.
References
- Abrams J.M., White K., Fessler L.I., Steller H. Programmed cell death during Drosophila embryogenesis. Development. 1993;117:29–43. - PubMed
- Aker M., Zimran A., Abrahamov A., Horowitz M., Matzner Y. Abnormal neutrophil chemotaxis in Gaucher disease. Br. J. Haematol. 1993;83:187–191. - PubMed
- Allen T.C. Pulmonary Langerhans cell histiocytosis and other pulmonary histiocytic diseases: a review. Arch. Pathol. Lab. Med. 2008;132:1171–1181. - PubMed
Publication types
MeSH terms
Substances
Related information
Grants and funding
LinkOut - more resources
Full Text Sources
Other Literature Sources
Medical
Molecular Biology Databases