Insights into Autism Spectrum Disorder Genomic Architecture and Biology from 71 Risk Loci
- PMID:26402605
- PMCID: PMC4624267
- DOI: 10.1016/j.neuron.2015.09.016
Insights into Autism Spectrum Disorder Genomic Architecture and Biology from 71 Risk Loci
Abstract
Analysis of de novo CNVs (dnCNVs) from the full Simons Simplex Collection (SSC) (N = 2,591 families) replicates prior findings of strong association with autism spectrum disorders (ASDs) and confirms six risk loci (1q21.1, 3q29, 7q11.23, 16p11.2, 15q11.2-13, and 22q11.2). The addition of published CNV data from the Autism Genome Project (AGP) and exome sequencing data from the SSC and the Autism Sequencing Consortium (ASC) shows that genes within small de novo deletions, but not within large dnCNVs, significantly overlap the high-effect risk genes identified by sequencing. Alternatively, large dnCNVs are found likely to contain multiple modest-effect risk genes. Overall, we find strong evidence that de novo mutations are associated with ASD apart from the risk for intellectual disability. Extending the transmission and de novo association test (TADA) to include small de novo deletions reveals 71 ASD risk loci, including 6 CNV regions (noted above) and 65 risk genes (FDR ≤ 0.1).
Copyright © 2015 Elsevier Inc. All rights reserved.
Figures
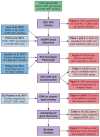
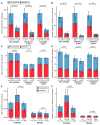
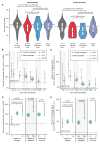
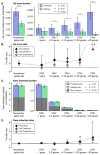
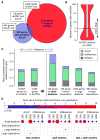
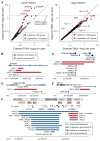
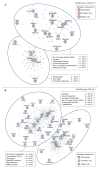
Similar articles
- Meta-Analyses Support Previous and Novel Autism Candidate Genes: Outcomes of an Unexplored Brazilian Cohort.da Silva Montenegro EM, Costa CS, Campos G, Scliar M, de Almeida TF, Zachi EC, Silva IMW, Chan AJS, Zarrei M, Lourenço NCV, Yamamoto GL, Scherer S, Passos-Bueno MR.da Silva Montenegro EM, et al.Autism Res. 2020 Feb;13(2):199-206. doi: 10.1002/aur.2238. Epub 2019 Nov 6.Autism Res. 2020.PMID:31696658
- Integrated Functional Analysis Implicates Syndromic and Rare Copy Number Variation Genes as Prominent Molecular Players in Pathogenesis of Autism Spectrum Disorders.Ashitha SNM, Ramachandra NB.Ashitha SNM, et al.Neuroscience. 2020 Jul 1;438:25-40. doi: 10.1016/j.neuroscience.2020.04.051. Epub 2020 May 12.Neuroscience. 2020.PMID:32407977
- Prevalence and phenotypic impact of rare potentially damaging variants in autism spectrum disorder.Mahjani B, De Rubeis S, Gustavsson Mahjani C, Mulhern M, Xu X, Klei L, Satterstrom FK, Fu J, Talkowski ME, Reichenberg A, Sandin S, Hultman CM, Grice DE, Roeder K, Devlin B, Buxbaum JD.Mahjani B, et al.Mol Autism. 2021 Oct 6;12(1):65. doi: 10.1186/s13229-021-00465-3.Mol Autism. 2021.PMID:34615535Free PMC article.
- Genetic architecture of autism spectrum disorder: Lessons from large-scale genomic studies.Choi L, An JY.Choi L, et al.Neurosci Biobehav Rev. 2021 Sep;128:244-257. doi: 10.1016/j.neubiorev.2021.06.028. Epub 2021 Jun 21.Neurosci Biobehav Rev. 2021.PMID:34166716Review.
- The genetics of autism.Muhle R, Trentacoste SV, Rapin I.Muhle R, et al.Pediatrics. 2004 May;113(5):e472-86. doi: 10.1542/peds.113.5.e472.Pediatrics. 2004.PMID:15121991Review.
Cited by
- The Pivotal Role of Aldehyde Toxicity in Autism Spectrum Disorder: The Therapeutic Potential of Micronutrient Supplementation.Jurnak F.Jurnak F.Nutr Metab Insights. 2016 Jun 14;8(Suppl 1):57-77. doi: 10.4137/NMI.S29531. eCollection 2015.Nutr Metab Insights. 2016.PMID:27330305Free PMC article.
- A Cre-dependent massively parallel reporter assay allows for cell-type specific assessment of the functional effects of non-coding elements in vivo.Lagunas T Jr, Plassmeyer SP, Fischer AD, Friedman RZ, Rieger MA, Selmanovic D, Sarafinovska S, Sol YK, Kasper MJ, Fass SB, Aguilar Lucero AF, An JY, Sanders SJ, Cohen BA, Dougherty JD.Lagunas T Jr, et al.Commun Biol. 2023 Nov 13;6(1):1151. doi: 10.1038/s42003-023-05483-w.Commun Biol. 2023.PMID:37953348Free PMC article.
- A neurogenetic analysis of female autism.Jack A, Sullivan CAW, Aylward E, Bookheimer SY, Dapretto M, Gaab N, Van Horn JD, Eilbott J, Jacokes Z, Torgerson CM, Bernier RA, Geschwind DH, McPartland JC, Nelson CA, Webb SJ, Pelphrey KA, Gupta AR; GENDAAR Consortium.Jack A, et al.Brain. 2021 Jul 28;144(6):1911-1926. doi: 10.1093/brain/awab064.Brain. 2021.PMID:33860292Free PMC article.
- The Genetic Control of Stoichiometry Underlying Autism.Darnell RB.Darnell RB.Annu Rev Neurosci. 2020 Jul 8;43:509-533. doi: 10.1146/annurev-neuro-100119-024851.Annu Rev Neurosci. 2020.PMID:32640929Free PMC article.
- Identification of a distinct developmental and behavioral profile in children with Dup15q syndrome.DiStefano C, Gulsrud A, Huberty S, Kasari C, Cook E, Reiter LT, Thibert R, Jeste SS.DiStefano C, et al.J Neurodev Disord. 2016 May 6;8:19. doi: 10.1186/s11689-016-9152-y. eCollection 2016.J Neurodev Disord. 2016.PMID:27158270Free PMC article.
References
- Berkel S, Marshall CR, Weiss B, Howe J, Roeth R, Moog U, Endris V, Roberts W, Szatmari P, Pinto D, et al. Mutations in the SHANK2 synaptic scaffolding gene in autism spectrum disorder and mental retardation. Nat Genet. 2010;42:489–491. - PubMed
Publication types
MeSH terms
Related information
Grants and funding
- MH19961-14/MH/NIMH NIH HHS/United States
- R01 MH074090/MH/NIMH NIH HHS/United States
- MH100233/MH/NIMH NIH HHS/United States
- R37 MH057881/MH/NIMH NIH HHS/United States
- R01 MH097849/MH/NIMH NIH HHS/United States
- MH100209/MH/NIMH NIH HHS/United States
- U01 MH100229/MH/NIMH NIH HHS/United States
- MH074090/MH/NIMH NIH HHS/United States
- R01 DC009410/DC/NIDCD NIH HHS/United States
- T32 MH019961/MH/NIMH NIH HHS/United States
- U01 MH100209/MH/NIMH NIH HHS/United States
- U01 MH100239/MH/NIMH NIH HHS/United States
- DC009410/DC/NIDCD NIH HHS/United States
- MH100239/MH/NIMH NIH HHS/United States
- MH100229/MH/NIMH NIH HHS/United States
- R01 MH057881/MH/NIMH NIH HHS/United States
- S10 OD018522/OD/NIH HHS/United States
- P30 DC005188/DC/NIDCD NIH HHS/United States
- Canadian Institutes of Health Research/Canada
- MH071584-07/MH/NIMH NIH HHS/United States
- R25 MH071584/MH/NIMH NIH HHS/United States
- T32 MH014276/MH/NIMH NIH HHS/United States
- Howard Hughes Medical Institute/United States
- MH057881/MH/NIMH NIH HHS/United States
- U01 MH100233/MH/NIMH NIH HHS/United States
LinkOut - more resources
Full Text Sources
Other Literature Sources
Medical
Miscellaneous