Coordinated Feeding Behavior in Trichoplax, an Animal without Synapses
- PMID:26333190
- PMCID: PMC4558020
- DOI: 10.1371/journal.pone.0136098
Coordinated Feeding Behavior in Trichoplax, an Animal without Synapses
Abstract
Trichoplax is a small disk-shaped marine metazoan that adheres to substrates and locomotes by ciliary gliding. Despite having only six cell types and lacking synapses Trichoplax coordinates a complex sequence of behaviors culminating in external digestion of algae. We combine live cell imaging with electron microscopy to show how this is accomplished. When Trichoplax glides over a patch of algae, its cilia stop beating so it ceases moving. A subset of one of the cell types, lipophils, simultaneously secretes granules whose content rapidly lyses algae. This secretion is accurately targeted, as only lipophils located near algae release granules. The animal pauses while the algal content is ingested, and then resumes gliding. Global control of gliding is coordinated with precise local control of lipophil secretion suggesting the presence of mechanisms for cellular communication and integration.
Conflict of interest statement
Figures
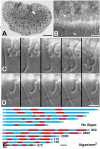
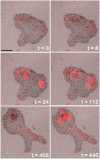
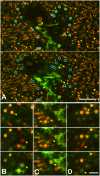
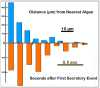
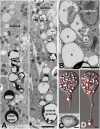
Similar articles
- Neuropeptidergic integration of behavior inTrichoplax adhaerens, an animal without synapses.Senatore A, Reese TS, Smith CL.Senatore A, et al.J Exp Biol. 2017 Sep 15;220(Pt 18):3381-3390. doi: 10.1242/jeb.162396.J Exp Biol. 2017.PMID:28931721Free PMC article.
- Coherent directed movement toward food modeled inTrichoplax, a ciliated animal lacking a nervous system.Smith CL, Reese TS, Govezensky T, Barrio RA.Smith CL, et al.Proc Natl Acad Sci U S A. 2019 Apr 30;116(18):8901-8908. doi: 10.1073/pnas.1815655116. Epub 2019 Apr 12.Proc Natl Acad Sci U S A. 2019.PMID:30979806Free PMC article.
- The ventral epithelium ofTrichoplax adhaerens deploys in distinct patterns cells that secrete digestive enzymes, mucus or diverse neuropeptides.Mayorova TD, Hammar K, Winters CA, Reese TS, Smith CL.Mayorova TD, et al.Biol Open. 2019 Aug 9;8(8):bio045674. doi: 10.1242/bio.045674.Biol Open. 2019.PMID:31366453Free PMC article.
- Insights into the evolution of digestive systems from studies of Trichoplax adhaerens.Smith CL, Mayorova TD.Smith CL, et al.Cell Tissue Res. 2019 Sep;377(3):353-367. doi: 10.1007/s00441-019-03057-z. Epub 2019 Jul 3.Cell Tissue Res. 2019.PMID:31270610Review.
- [From Vendian to Cambrian: the beginning of morphological disparity of modern Metazoan phyla].Rozhnov SV.Rozhnov SV.Ontogenez. 2010 Nov-Dec;41(6):425-37.Ontogenez. 2010.PMID:21268365Review.Russian.
Cited by
- The Diversity of Spine Synapses in Animals.Petralia RS, Wang YX, Mattson MP, Yao PJ.Petralia RS, et al.Neuromolecular Med. 2016 Dec;18(4):497-539. doi: 10.1007/s12017-016-8405-y. Epub 2016 May 26.Neuromolecular Med. 2016.PMID:27230661Free PMC article.Review.
- Placozoan secretory cell types implicated in feeding, innate immunity and regulation of behavior.Mayorova TD, Koch TL, Kachar B, Jung JH, Reese TS, Smith CL.Mayorova TD, et al.bioRxiv [Preprint]. 2025 Jan 16:2024.09.18.613768. doi: 10.1101/2024.09.18.613768.bioRxiv. 2025.PMID:39372748Free PMC article.Preprint.
- Neuronal coordination of motile cilia in locomotion and feeding.Marinković M, Berger J, Jékely G.Marinković M, et al.Philos Trans R Soc Lond B Biol Sci. 2020 Feb 17;375(1792):20190165. doi: 10.1098/rstb.2019.0165. Epub 2019 Dec 30.Philos Trans R Soc Lond B Biol Sci. 2020.PMID:31884921Free PMC article.Review.
- Function and phylogeny support the independent evolution of an ASIC-like Deg/ENaC channel in the Placozoa.Elkhatib W, Yanez-Guerra LA, Mayorova TD, Currie MA, Singh A, Perera M, Gauberg J, Senatore A.Elkhatib W, et al.Commun Biol. 2023 Sep 18;6(1):951. doi: 10.1038/s42003-023-05312-0.Commun Biol. 2023.PMID:37723223Free PMC article.
- Amino acids integrate behaviors in nerveless placozoans.Nikitin MA, Romanova DY, Borman SI, Moroz LL.Nikitin MA, et al.Front Neurosci. 2023 Apr 13;17:1125624. doi: 10.3389/fnins.2023.1125624. eCollection 2023.Front Neurosci. 2023.PMID:37123368Free PMC article.
References
- Schulze FE. Uber Trichoplax adhaerens. Phys Abh Kgl Acad Wiss Berl. 1891;1–23.
- Grell KG, Ruthmann A. Placozoa In: Harrison FW, Westfall JA, editors. Microscopic Anatomy of Invertebrates. New York: Wiley-Liss; 1991. p. 13–27.
- Signorovitch AY, Dellaporta SL, Buss LW. Caribbean Placozoan Phylogeography. Biol Bull. 2006. October 1;211(2):149–56. - PubMed
Publication types
MeSH terms
Grants and funding
LinkOut - more resources
Full Text Sources
Other Literature Sources