Neuroimaging and neuromodulation approaches to study eating behavior and prevent and treat eating disorders and obesity
- PMID:26110109
- PMCID: PMC4473270
- DOI: 10.1016/j.nicl.2015.03.016
Neuroimaging and neuromodulation approaches to study eating behavior and prevent and treat eating disorders and obesity
Abstract
Functional, molecular and genetic neuroimaging has highlighted the existence of brain anomalies and neural vulnerability factors related to obesity and eating disorders such as binge eating or anorexia nervosa. In particular, decreased basal metabolism in the prefrontal cortex and striatum as well as dopaminergic alterations have been described in obese subjects, in parallel with increased activation of reward brain areas in response to palatable food cues. Elevated reward region responsivity may trigger food craving and predict future weight gain. This opens the way to prevention studies using functional and molecular neuroimaging to perform early diagnostics and to phenotype subjects at risk by exploring different neurobehavioral dimensions of the food choices and motivation processes. In the first part of this review, advantages and limitations of neuroimaging techniques, such as functional magnetic resonance imaging (fMRI), positron emission tomography (PET), single photon emission computed tomography (SPECT), pharmacogenetic fMRI and functional near-infrared spectroscopy (fNIRS) will be discussed in the context of recent work dealing with eating behavior, with a particular focus on obesity. In the second part of the review, non-invasive strategies to modulate food-related brain processes and functions will be presented. At the leading edge of non-invasive brain-based technologies is real-time fMRI (rtfMRI) neurofeedback, which is a powerful tool to better understand the complexity of human brain-behavior relationships. rtfMRI, alone or when combined with other techniques and tools such as EEG and cognitive therapy, could be used to alter neural plasticity and learned behavior to optimize and/or restore healthy cognition and eating behavior. Other promising non-invasive neuromodulation approaches being explored are repetitive transcranial magnetic stimulation (rTMS) and transcranial direct-current stimulation (tDCS). Converging evidence points at the value of these non-invasive neuromodulation strategies to study basic mechanisms underlying eating behavior and to treat its disorders. Both of these approaches will be compared in light of recent work in this field, while addressing technical and practical questions. The third part of this review will be dedicated to invasive neuromodulation strategies, such as vagus nerve stimulation (VNS) and deep brain stimulation (DBS). In combination with neuroimaging approaches, these techniques are promising experimental tools to unravel the intricate relationships between homeostatic and hedonic brain circuits. Their potential as additional therapeutic tools to combat pharmacorefractory morbid obesity or acute eating disorders will be discussed, in terms of technical challenges, applicability and ethics. In a general discussion, we will put the brain at the core of fundamental research, prevention and therapy in the context of obesity and eating disorders. First, we will discuss the possibility to identify new biological markers of brain functions. Second, we will highlight the potential of neuroimaging and neuromodulation in individualized medicine. Third, we will introduce the ethical questions that are concomitant to the emergence of new neuromodulation therapies.
Keywords: 5-HT, serotonin; ADHD, attention deficit hyperactivity disorder; AN, anorexia nervosa; ANT, anterior nucleus of the thalamus; B N, bulimia nervosa; BAT, brown adipose tissue; BED, binge eating disorder; BMI, body mass index; BOLD, blood oxygenation level dependent; BS, bariatric surgery; Brain; CBF, cerebral blood flow; CCK, cholecystokinin; Cg25, subgenual cingulate cortex; DA, dopamine; DAT, dopamine transporter; DBS, deep brain stimulation; DBT, deep brain therapy; DTI, diffusion tensor imaging; ED, eating disorders; EEG, electroencephalography; Eating disorders; GP, globus pallidus; HD-tDCS, high-definition transcranial direct current stimulation; HFD, high-fat diet; HHb, deoxygenated-hemoglobin; Human; LHA, lateral hypothalamus; MER, microelectrode recording; MRS, magnetic resonance spectroscopy; Nac, nucleus accumbens; Neuroimaging; Neuromodulation; O2Hb, oxygenated-hemoglobin; OCD, obsessive–compulsive disorder; OFC, orbitofrontal cortex; Obesity; PD, Parkinson's disease; PET, positron emission tomography; PFC, prefrontal cortex; PYY, peptide tyrosine tyrosine; SPECT, single photon emission computed tomography; STN, subthalamic nucleus; TMS, transcranial magnetic stimulation; TRD, treatment-resistant depression; VBM, voxel-based morphometry; VN, vagus nerve; VNS, vagus nerve stimulation; VS, ventral striatum; VTA, ventral tegmental area; aCC, anterior cingulate cortex; dTMS, deep transcranial magnetic stimulation; daCC, dorsal anterior cingulate cortex; dlPFC, dorsolateral prefrontal cortex; fMRI, functional magnetic resonance imaging; fNIRS, functional near-infrared spectroscopy; lPFC, lateral prefrontal cortex; pCC, posterior cingulate cortex; rCBF, regional cerebral blood flow; rTMS, repetitive transcranial magnetic stimulation; rtfMRI, real-time functional magnetic resonance imaging; tACS, transcranial alternate current stimulation; tDCS, transcranial direct current stimulation; tRNS, transcranial random noise stimulation; vlPFC, ventrolateral prefrontal cortex; vmH, ventromedial hypothalamus; vmPFC, ventromedial prefrontal cortex.
Figures
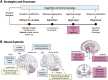
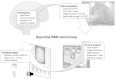
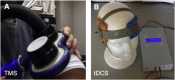
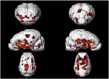
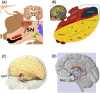
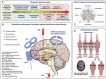
Similar articles
- A systematic review of the effects of neuromodulation on eating and body weight: evidence from human and animal studies.McClelland J, Bozhilova N, Campbell I, Schmidt U.McClelland J, et al.Eur Eat Disord Rev. 2013 Nov;21(6):436-55. doi: 10.1002/erv.2256. Epub 2013 Sep 20.Eur Eat Disord Rev. 2013.PMID:24155246Review.
- Factors modulating neural reactivity to drug cues in addiction: a survey of human neuroimaging studies.Jasinska AJ, Stein EA, Kaiser J, Naumer MJ, Yalachkov Y.Jasinska AJ, et al.Neurosci Biobehav Rev. 2014 Jan;38:1-16. doi: 10.1016/j.neubiorev.2013.10.013. Epub 2013 Nov 6.Neurosci Biobehav Rev. 2014.PMID:24211373Free PMC article.Review.
- [Neuromodulation as an intervention for addiction: overview and future prospects].Luigjes J, Breteler R, Vanneste S, de Ridder D.Luigjes J, et al.Tijdschr Psychiatr. 2013;55(11):841-52.Tijdschr Psychiatr. 2013.PMID:24242143Review.Dutch.
- Role of the dorsal anterior cingulate cortex in obsessive-compulsive disorder: converging evidence from cognitive neuroscience and psychiatric neurosurgery.McGovern RA, Sheth SA.McGovern RA, et al.J Neurosurg. 2017 Jan;126(1):132-147. doi: 10.3171/2016.1.JNS15601. Epub 2016 Apr 1.J Neurosurg. 2017.PMID:27035167
- Increases in frontostriatal connectivity are associated with response to dorsomedial repetitive transcranial magnetic stimulation in refractory binge/purge behaviors.Dunlop K, Woodside B, Lam E, Olmsted M, Colton P, Giacobbe P, Downar J.Dunlop K, et al.Neuroimage Clin. 2015 Jul 2;8:611-8. doi: 10.1016/j.nicl.2015.06.008. eCollection 2015.Neuroimage Clin. 2015.PMID:26199873Free PMC article.
Cited by
- Differences in cortical microstructure according to body mass index in neurologically healthy populations using structural magnetic resonance imaging.Park Y, Namgung JY, Kim CY, Park Y, Park BY.Park Y, et al.Heliyon. 2024 Jun 15;10(12):e33134. doi: 10.1016/j.heliyon.2024.e33134. eCollection 2024 Jun 30.Heliyon. 2024.PMID:38984310Free PMC article.
- Clinical Utility of Add-On Transcranial Direct Current Stimulation for Binge Eating Disorder with Obesity in Schizophrenia.Sreeraj VS, Masali M, Shivakumar V, Bose A, Venkatasubramanian G.Sreeraj VS, et al.Indian J Psychol Med. 2018 Sep-Oct;40(5):487-490. doi: 10.4103/IJPSYM.IJPSYM_551_17.Indian J Psychol Med. 2018.PMID:30275626Free PMC article.
- Efficacy and acceptability of noninvasive brain stimulation interventions for weight reduction in obesity: a pilot network meta-analysis.Zeng BY, Zeng BS, Chen YW, Hung CM, Sun CK, Cheng YS, Stubbs B, Carvalho AF, Brunoni AR, Su KP, Tu YK, Wu YC, Chen TY, Lin PY, Liang CS, Hsu CW, Tseng PT, Li CT.Zeng BY, et al.Int J Obes (Lond). 2021 Aug;45(8):1705-1716. doi: 10.1038/s41366-021-00833-2. Epub 2021 May 10.Int J Obes (Lond). 2021.PMID:33972697
- High body mass index, brain metabolism and connectivity: an unfavorable effect in elderly females.Sala A, Malpetti M, Ferrulli A, Gianolli L, Luzi L, Perani D; Alzheimer’s Disease Neuroimaging Initiative (ADNI) database.Sala A, et al.Aging (Albany NY). 2019 Oct 9;11(19):8573-8586. doi: 10.18632/aging.102347. Epub 2019 Oct 9.Aging (Albany NY). 2019.PMID:31600734Free PMC article.
- EnhancingDLG2 Implications in Neuropsychiatric Disorders: Analysis of a Cohort of Eight Patients with 11q14.1 Imbalances.Bertini V, Milone R, Cristofani P, Cambi F, Bosetti C, Barbieri F, Bertelloni S, Cioni G, Valetto A, Battini R.Bertini V, et al.Genes (Basel). 2022 May 12;13(5):859. doi: 10.3390/genes13050859.Genes (Basel). 2022.PMID:35627244Free PMC article.
References
- Adams T.D., Davidson L.E., Litwin S.E., Kolotkin R.L., LaMonte M.J., Pendleton R.C., Strong M.B., Vinik R., Wanner N.A., Hopkins P.N., Gress R.E., Walker J.M., Cloward T.V., Nuttall R.T., Hammoud A., Greenwood J.L., Crosby R.D., McKinlay R., Simper S.C., Smith S.C. Health benefits of gastric bypass surgery after 6 years. JAMA. 2012;308(11):1122–1131.22990271 - PMC - PubMed
- Adcock R.A., Lutomski K., Mcleod S.R., Soneji D.J., Gabrieli J.D. Real-time fMRI during the psychotherapy session: toward a methodology to augment therapeutic benefit, exemplary data. 2005. Human Brain Mapping Conference.
- Aerts H.J., Velazquez E.R., Leijenaar R.T., Parmar C., Grossmann P., Cavalho S., Bussink J., Monshouwer R., Haibe-Kains B., Rietveld D., Hoebers F., Rietbergen M.M., Leemans C.R., Dekker A., Quackenbush J., Gillies R.J., Lambin P. Decoding tumour phenotype by noninvasive imaging using a quantitative radiomics approach. Nat. Commun. 2014;5:4006.24892406 - PMC - PubMed
Publication types
MeSH terms
Related information
Grants and funding
LinkOut - more resources
Full Text Sources
Other Literature Sources
Medical
Miscellaneous