Targeting mitochondria metabolism for cancer therapy
- PMID:25517383
- PMCID: PMC4340667
- DOI: 10.1038/nchembio.1712
Targeting mitochondria metabolism for cancer therapy
Abstract
Mitochondria have a well-recognized role in the production of ATP and the intermediates needed for macromolecule biosynthesis, such as nucleotides. Mitochondria also participate in the activation of signaling pathways. Overall, accumulating evidence now suggests that mitochondrial bioenergetics, biosynthesis and signaling are required for tumorigenesis. Thus, emerging studies have begun to demonstrate that mitochondrial metabolism is potentially a fruitful arena for cancer therapy. In this Perspective, we highlight recent developments in targeting mitochondrial metabolism for the treatment of cancer.
Conflict of interest statement
The authors declare competing financial interests: details accompany the online version of the paper.
Figures
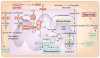
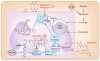
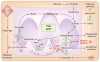
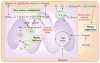
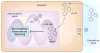
Similar articles
- Mitochondria and cancer chemoresistance.Guerra F, Arbini AA, Moro L.Guerra F, et al.Biochim Biophys Acta Bioenerg. 2017 Aug;1858(8):686-699. doi: 10.1016/j.bbabio.2017.01.012. Epub 2017 Feb 1.Biochim Biophys Acta Bioenerg. 2017.PMID:28161329Review.
- Restoration of mitochondria function as a target for cancer therapy.Bhat TA, Kumar S, Chaudhary AK, Yadav N, Chandra D.Bhat TA, et al.Drug Discov Today. 2015 May;20(5):635-43. doi: 10.1016/j.drudis.2015.03.001. Epub 2015 Mar 9.Drug Discov Today. 2015.PMID:25766095Free PMC article.Review.
- A review of the basics of mitochondrial bioenergetics, metabolism, and related signaling pathways in cancer cells: Therapeutic targeting of tumor mitochondria with lipophilic cationic compounds.Kalyanaraman B, Cheng G, Hardy M, Ouari O, Lopez M, Joseph J, Zielonka J, Dwinell MB.Kalyanaraman B, et al.Redox Biol. 2018 Apr;14:316-327. doi: 10.1016/j.redox.2017.09.020. Epub 2017 Sep 29.Redox Biol. 2018.PMID:29017115Free PMC article.Review.
- Targeting mitochondria in the treatment of human cancer: a coordinated attack against cancer cell energy metabolism and signalling.Hagland H, Nikolaisen J, Hodneland LI, Gjertsen BT, Bruserud Ø, Tronstad KJ.Hagland H, et al.Expert Opin Ther Targets. 2007 Aug;11(8):1055-69. doi: 10.1517/14728222.11.8.1055.Expert Opin Ther Targets. 2007.PMID:17665978Review.
- Tumor cell metabolism: cancer's Achilles' heel.Kroemer G, Pouyssegur J.Kroemer G, et al.Cancer Cell. 2008 Jun;13(6):472-82. doi: 10.1016/j.ccr.2008.05.005.Cancer Cell. 2008.PMID:18538731Review.
Cited by
- Mitochondria in epithelial ovarian carcinoma exhibit abnormal phenotypes and blunted associations with biobehavioral factors.Bindra S, McGill MA, Triplett MK, Tyagi A, Thaker PH, Dahmoush L, Goodheart MJ, Ogden RT, Owusu-Ansah E, R Karan K, Cole S, Sood AK, Lutgendorf SK, Picard M.Bindra S, et al.Sci Rep. 2021 Jun 2;11(1):11595. doi: 10.1038/s41598-021-89934-6.Sci Rep. 2021.PMID:34078919Free PMC article.
- Preventative and Therapeutic Effects of Metformin in Gastric Cancer: A New Contribution of an Old Friend.Zhang J, Wen L, Zhou Q, He K, Teng L.Zhang J, et al.Cancer Manag Res. 2020 Sep 16;12:8545-8554. doi: 10.2147/CMAR.S264032. eCollection 2020.Cancer Manag Res. 2020.PMID:32982447Free PMC article.Review.
- Assessment of Betulinic Acid Cytotoxicity and Mitochondrial Metabolism Impairment in a Human Melanoma Cell Line.Coricovac D, Dehelean CA, Pinzaru I, Mioc A, Aburel OM, Macasoi I, Draghici GA, Petean C, Soica C, Boruga M, Vlaicu B, Muntean MD.Coricovac D, et al.Int J Mol Sci. 2021 May 4;22(9):4870. doi: 10.3390/ijms22094870.Int J Mol Sci. 2021.PMID:34064489Free PMC article.
- Inhibition of mitochondrial translocase SLC25A5 and histone deacetylation is an effective combination therapy in neuroblastoma.Seneviratne JA, Carter DR, Mittra R, Gifford A, Kim PY, Luo JS, Mayoh C, Salib A, Rahmanto AS, Murray J, Cheng NC, Nagy Z, Wang Q, Kleynhans A, Tan O, Sutton SK, Xue C, Chung SA, Zhang Y, Sun C, Zhang L, Haber M, Norris MD, Fletcher JI, Liu T, Dilda PJ, Hogg PJ, Cheung BB, Marshall GM.Seneviratne JA, et al.Int J Cancer. 2023 Apr 1;152(7):1399-1413. doi: 10.1002/ijc.34349. Epub 2022 Nov 17.Int J Cancer. 2023.PMID:36346110Free PMC article.
- AMPK activity regulates trafficking of mitochondria to the leading edge during cell migration and matrix invasion.Cunniff B, McKenzie AJ, Heintz NH, Howe AK.Cunniff B, et al.Mol Biol Cell. 2016 Sep 1;27(17):2662-74. doi: 10.1091/mbc.E16-05-0286. Epub 2016 Jul 6.Mol Biol Cell. 2016.PMID:27385336Free PMC article.
References
- Bonnet S, et al. A mitochondria-K channel axis is suppressed in cancer and its normalization promotes apoptosis and inhibits cancer growth. Cancer Cell. 2007;11:37–51. - PubMed
- Warburg O. On the origin of cancer cells. Science. 1956;123:309–314. - PubMed
- Koppenol WH, Bounds PL, Dang CV. Otto Warburg’s contributions to current concepts of cancer metabolism. Nat Rev Cancer. 2011;11:325–337. - PubMed
- Cross CE, et al. Oxygen radicals and human disease. Ann Intern Med. 1987;107:526–545. - PubMed
Publication types
MeSH terms
Substances
Related information
Grants and funding
LinkOut - more resources
Full Text Sources
Other Literature Sources