Investigations of the efficient electrocatalytic interconversions of carbon dioxide and carbon monoxide by nickel-containing carbon monoxide dehydrogenases
- PMID:25416391
- PMCID: PMC4261625
- DOI: 10.1007/978-94-017-9269-1_4
Investigations of the efficient electrocatalytic interconversions of carbon dioxide and carbon monoxide by nickel-containing carbon monoxide dehydrogenases
Abstract
Carbon monoxide dehydrogenases (CODH) play an important role in utilizing carbon monoxide (CO) or carbon dioxide (CO2) in the metabolism of some microorganisms. Two distinctly different types of CODH are distinguished by the elements constituting the active site. A Mo-Cu containing CODH is found in some aerobic organisms, whereas a Ni-Fe containing CODH (henceforth simply Ni-CODH) is found in some anaerobes. Two members of the simplest class (IV) of Ni-CODH behave as efficient, reversible electrocatalysts of CO2/CO interconversion when adsorbed on a graphite electrode. Their intense electroactivity sets an important benchmark for the standard of performance at which synthetic molecular and material electrocatalysts comprised of suitably attired abundant first-row transition elements must be able to operate. Investigations of CODHs by protein film electrochemistry (PFE) reveal how the enzymes respond to the variable electrode potential that can drive CO2/CO interconversion in each direction, and identify the potential thresholds at which different small molecules, both substrates and inhibitors, enter or leave the catalytic cycle. Experiments carried out on a much larger (Class III) enzyme CODH/ACS, in which CODH is complexed tightly with acetyl-CoA synthase, show that some of these characteristics are retained, albeit with much slower rates of interfacial electron transfer, attributable to the difficulty in making good electronic contact at the electrode. The PFE results complement and clarify investigations made using spectroscopic investigations.
Figures
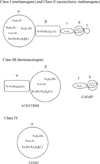
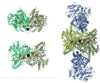
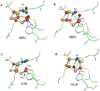
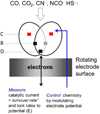
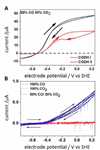
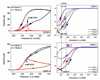
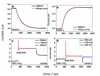
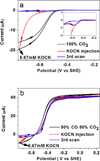
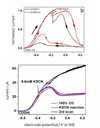
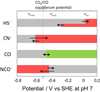
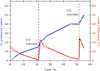
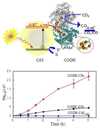
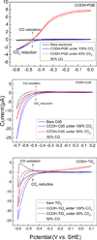
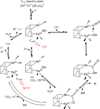
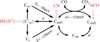
Similar articles
- O2 Inhibition of Ni-Containing CO Dehydrogenase Is Partly Reversible.Merrouch M, Hadj-Saïd J, Domnik L, Dobbek H, Léger C, Dementin S, Fourmond V.Merrouch M, et al.Chemistry. 2015 Dec 21;21(52):18934-8. doi: 10.1002/chem.201502835. Epub 2015 Nov 16.Chemistry. 2015.PMID:26568460
- X-Ray Crystallography of Carbon Monoxide Dehydrogenases.Jeoung JH, Martins BM, Dobbek H.Jeoung JH, et al.Methods Mol Biol. 2019;1876:167-178. doi: 10.1007/978-1-4939-8864-8_11.Methods Mol Biol. 2019.PMID:30317481
- Carbon monoxide. Toxic gas and fuel for anaerobes and aerobes: carbon monoxide dehydrogenases.Jeoung JH, Fesseler J, Goetzl S, Dobbek H.Jeoung JH, et al.Met Ions Life Sci. 2014;14:37-69. doi: 10.1007/978-94-017-9269-1_3.Met Ions Life Sci. 2014.PMID:25416390Review.
- Investigations of two bidirectional carbon monoxide dehydrogenases from Carboxydothermus hydrogenoformans by protein film electrochemistry.Wang VC, Ragsdale SW, Armstrong FA.Wang VC, et al.Chembiochem. 2013 Sep 23;14(14):1845-51. doi: 10.1002/cbic.201300270. Epub 2013 Sep 3.Chembiochem. 2013.PMID:24002936Free PMC article.
- A role for nickel-iron cofactors in biological carbon monoxide and carbon dioxide utilization.Kung Y, Drennan CL.Kung Y, et al.Curr Opin Chem Biol. 2011 Apr;15(2):276-83. doi: 10.1016/j.cbpa.2010.11.005. Epub 2010 Dec 2.Curr Opin Chem Biol. 2011.PMID:21130022Free PMC article.Review.
Cited by
- Ligand binding at the A-cluster in full-length or truncated acetyl-CoA synthase studied by X-ray absorption spectroscopy.Schrapers P, Ilina J, Gregg CM, Mebs S, Jeoung JH, Dau H, Dobbek H, Haumann M.Schrapers P, et al.PLoS One. 2017 Feb 8;12(2):e0171039. doi: 10.1371/journal.pone.0171039. eCollection 2017.PLoS One. 2017.PMID:28178309Free PMC article.
- Electronic isomerism in a heterometallic nickel-iron-sulfur cluster models substrate binding and cyanide inhibition of carbon monoxide dehydrogenase.Lewis LC, Sanabria-Gracia JA, Lee Y, Jenkins AJ, Shafaat HS.Lewis LC, et al.Chem Sci. 2024 Mar 27;15(16):5916-5928. doi: 10.1039/d4sc00023d. eCollection 2024 Apr 24.Chem Sci. 2024.PMID:38665523Free PMC article.
- Current status of carbon monoxide dehydrogenases (CODH) and their potential for electrochemical applications.Bährle R, Böhnke S, Englhard J, Bachmann J, Perner M.Bährle R, et al.Bioresour Bioprocess. 2023 Nov 27;10(1):84. doi: 10.1186/s40643-023-00705-9.Bioresour Bioprocess. 2023.PMID:38647803Free PMC article.Review.
- Bio-inspired CO2 reduction by a rhenium tricarbonyl bipyridine-based catalyst appended to amino acids and peptidic platforms: incorporating proton relays and hydrogen-bonding functional groups.Chabolla SA, Machan CW, Yin J, Dellamary EA, Sahu S, Gianneschi NC, Gilson MK, Tezcan FA, Kubiak CP.Chabolla SA, et al.Faraday Discuss. 2017 Jun 2;198:279-300. doi: 10.1039/c7fd00003k.Faraday Discuss. 2017.PMID:28374024Free PMC article.
References
- Ferry JG. How to Make a Living by Exhaling Methane. Annual Review of Microbiology, Vol 64, 2010. 2010;64:453–473. - PubMed
- Oelgeschlaeger E, Rother M. Carbon monoxide-dependent energy metabolism in anaerobic bacteria and archaea. Archives of Microbiology. 2008;190(3):257–269. - PubMed
- Meyer O, Schlegel HG. BIOLOGY OF AEROBIC CARBON-MONOXIDE OXIDIZING BACTERIA. Annual Review of Microbiology. 1983;37:277–310. - PubMed
Publication types
MeSH terms
Substances
Related information
Grants and funding
LinkOut - more resources
Full Text Sources
Other Literature Sources