Intrinsically disordered segments affect protein half-life in the cell and during evolution
- PMID:25220455
- PMCID: PMC4358326
- DOI: 10.1016/j.celrep.2014.07.055
Intrinsically disordered segments affect protein half-life in the cell and during evolution
Abstract
Precise control of protein turnover is essential for cellular homeostasis. The ubiquitin-proteasome system is well established as a major regulator of protein degradation, but an understanding of how inherent structural features influence the lifetimes of proteins is lacking. We report that yeast, mouse, and human proteins with terminal or internal intrinsically disordered segments have significantly shorter half-lives than proteins without these features. The lengths of the disordered segments that affect protein half-life are compatible with the structure of the proteasome. Divergence in terminal and internal disordered segments in yeast proteins originating from gene duplication leads to significantly altered half-life. Many paralogs that are affected by such changes participate in signaling, where altered protein half-life will directly impact cellular processes and function. Thus, natural variation in the length and position of disordered segments may affect protein half-life and could serve as an underappreciated source of genetic variation with important phenotypic consequences.
Copyright © 2014 The Authors. Published by Elsevier Inc. All rights reserved.
Figures
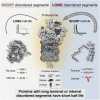
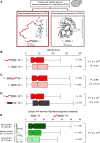
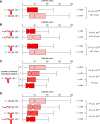
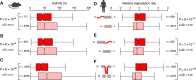
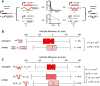
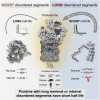
Similar articles
- Sequence composition of disordered regions fine-tunes protein half-life.Fishbain S, Inobe T, Israeli E, Chavali S, Yu H, Kago G, Babu MM, Matouschek A.Fishbain S, et al.Nat Struct Mol Biol. 2015 Mar;22(3):214-21. doi: 10.1038/nsmb.2958. Epub 2015 Feb 2.Nat Struct Mol Biol. 2015.PMID:25643324Free PMC article.
- Genome-scale molecular principles of mRNA half-life regulation in yeast.Basu S, Mallik S, Hait S, Kundu S.Basu S, et al.FEBS J. 2021 Jun;288(11):3428-3447. doi: 10.1111/febs.15670. Epub 2021 Jan 8.FEBS J. 2021.PMID:33319437
- System-wide analysis reveals intrinsically disordered proteins are prone to ubiquitylation after misfolding stress.Ng AH, Fang NN, Comyn SA, Gsponer J, Mayor T.Ng AH, et al.Mol Cell Proteomics. 2013 Sep;12(9):2456-67. doi: 10.1074/mcp.M112.023416. Epub 2013 May 28.Mol Cell Proteomics. 2013.PMID:23716602Free PMC article.
- How a disordered ubiquitin ligase maintains order in nuclear protein homeostasis.Rosenbaum JC, Gardner RG.Rosenbaum JC, et al.Nucleus. 2011 Jul-Aug;2(4):264-70. doi: 10.4161/nucl.2.4.16118. Epub 2011 Jul 1.Nucleus. 2011.PMID:21941105Free PMC article.Review.
- Protein dynamics and conformational disorder in molecular recognition.Mittag T, Kay LE, Forman-Kay JD.Mittag T, et al.J Mol Recognit. 2010 Mar-Apr;23(2):105-16. doi: 10.1002/jmr.961.J Mol Recognit. 2010.PMID:19585546Review.
Cited by
- An Inducible System for Rapid Degradation of Specific Cellular Proteins Using Proteasome Adaptors.Wilmington SR, Matouschek A.Wilmington SR, et al.PLoS One. 2016 Apr 4;11(4):e0152679. doi: 10.1371/journal.pone.0152679. eCollection 2016.PLoS One. 2016.PMID:27043013Free PMC article.
- The C-Terminus of the PSMA3 Proteasome Subunit Preferentially Traps Intrinsically Disordered Proteins for Degradation.Biran A, Myers N, Steinberger S, Adler J, Riutin M, Broennimann K, Reuven N, Shaul Y.Biran A, et al.Cells. 2022 Oct 14;11(20):3231. doi: 10.3390/cells11203231.Cells. 2022.PMID:36291102Free PMC article.
- Regulation of SETD2 stability is important for the fidelity of H3K36me3 deposition.Bhattacharya S, Workman JL.Bhattacharya S, et al.Epigenetics Chromatin. 2020 Oct 6;13(1):40. doi: 10.1186/s13072-020-00362-8.Epigenetics Chromatin. 2020.PMID:33023640Free PMC article.
- A repetitive acidic region contributes to the extremely rapid degradation of the cell-context essential protein TRIM52.Hacker K, Benke S, Agerer B, Scinicariello S, Budroni V, Versteeg GA.Hacker K, et al.Sci Rep. 2019 May 27;9(1):7901. doi: 10.1038/s41598-019-44359-0.Sci Rep. 2019.PMID:31133683Free PMC article.
- EPLIN-β is a novel substrate of ornithine decarboxylase antizyme 1 and mediates cellular migration.Li D, Neo SP, Gunaratne J, Sabapathy K.Li D, et al.J Cell Sci. 2023 Jun 15;136(12):jcs260427. doi: 10.1242/jcs.260427. Epub 2023 Jun 16.J Cell Sci. 2023.PMID:37325974Free PMC article.
References
- Altschul S.F., Gish W., Miller W., Myers E.W., Lipman D.J. Basic local alignment search tool. J. Mol. Biol. 1990;215:403–410. - PubMed
- Babon J.J., McManus E.J., Yao S., DeSouza D.P., Mielke L.A., Sprigg N.S., Willson T.A., Hilton D.J., Nicola N.A., Baca M. The structure of SOCS3 reveals the basis of the extended SH2 domain function and identifies an unstructured insertion that regulates stability. Mol. Cell. 2006;22:205–216. - PubMed
- Babu M.M., van der Lee R., de Groot N.S., Gsponer J. Intrinsically disordered proteins: regulation and disease. Curr. Opin. Struct. Biol. 2011;21:432–440. - PubMed
- Babu M.M., Kriwacki R.W., Pappu R.V. Structural biology. Versatility from protein disorder. Science. 2012;337:1460–1461. - PubMed
Publication types
MeSH terms
Substances
Grants and funding
LinkOut - more resources
Full Text Sources
Other Literature Sources
Molecular Biology Databases