Highly efficient CRISPR/Cas9-mediated knock-in in zebrafish by homology-independent DNA repair
- PMID:24179142
- PMCID: PMC3875856
- DOI: 10.1101/gr.161638.113
Highly efficient CRISPR/Cas9-mediated knock-in in zebrafish by homology-independent DNA repair
Abstract
Sequence-specific nucleases like TALENs and the CRISPR/Cas9 system have greatly expanded the genome editing possibilities in model organisms such as zebrafish. Both systems have recently been used to create knock-out alleles with great efficiency, and TALENs have also been successfully employed in knock-in of DNA cassettes at defined loci via homologous recombination (HR). Here we report CRISPR/Cas9-mediated knock-in of DNA cassettes into the zebrafish genome at a very high rate by homology-independent double-strand break (DSB) repair pathways. After co-injection of a donor plasmid with a short guide RNA (sgRNA) and Cas9 nuclease mRNA, concurrent cleavage of donor plasmid DNA and the selected chromosomal integration site resulted in efficient targeted integration of donor DNA. We successfully employed this approach to convert eGFP into Gal4 transgenic lines, and the same plasmids and sgRNAs can be applied in any species where eGFP lines were generated as part of enhancer and gene trap screens. In addition, we show the possibility of easily targeting DNA integration at endogenous loci, thus greatly facilitating the creation of reporter and loss-of-function alleles. Due to its simplicity, flexibility, and very high efficiency, our method greatly expands the repertoire for genome editing in zebrafish and can be readily adapted to many other organisms.
Figures
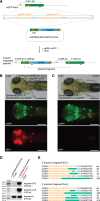
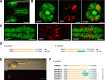
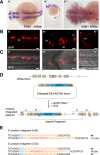
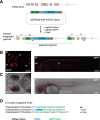
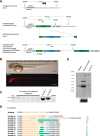
Similar articles
- Homology-Independent Integration of Plasmid DNA into the Zebrafish Genome.Auer TO, Del Bene F.Auer TO, et al.Methods Mol Biol. 2016;1451:31-51. doi: 10.1007/978-1-4939-3771-4_3.Methods Mol Biol. 2016.PMID:27464799
- Efficient targeted integration directed by short homology in zebrafish and mammalian cells.Wierson WA, Welker JM, Almeida MP, Mann CM, Webster DA, Torrie ME, Weiss TJ, Kambakam S, Vollbrecht MK, Lan M, McKeighan KC, Levey J, Ming Z, Wehmeier A, Mikelson CS, Haltom JA, Kwan KM, Chien CB, Balciunas D, Ekker SC, Clark KJ, Webber BR, Moriarity BS, Solin SL, Carlson DF, Dobbs DL, McGrail M, Essner J.Wierson WA, et al.Elife. 2020 May 15;9:e53968. doi: 10.7554/eLife.53968.Elife. 2020.PMID:32412410Free PMC article.
- Genome editing using CRISPR/Cas9-based knock-in approaches in zebrafish.Albadri S, Del Bene F, Revenu C.Albadri S, et al.Methods. 2017 May 15;121-122:77-85. doi: 10.1016/j.ymeth.2017.03.005. Epub 2017 Mar 12.Methods. 2017.PMID:28300641Review.
- Efficient generation of knock-in transgenic zebrafish carrying reporter/driver genes by CRISPR/Cas9-mediated genome engineering.Kimura Y, Hisano Y, Kawahara A, Higashijima S.Kimura Y, et al.Sci Rep. 2014 Oct 8;4:6545. doi: 10.1038/srep06545.Sci Rep. 2014.PMID:25293390Free PMC article.
- Zebrafish Genome Engineering Using the CRISPR-Cas9 System.Li M, Zhao L, Page-McCaw PS, Chen W.Li M, et al.Trends Genet. 2016 Dec;32(12):815-827. doi: 10.1016/j.tig.2016.10.005. Epub 2016 Nov 8.Trends Genet. 2016.PMID:27836208Free PMC article.Review.
Cited by
- CRISPR-Cas9-mediated single-gene and gene family disruption in Trypanosoma cruzi.Peng D, Kurup SP, Yao PY, Minning TA, Tarleton RL.Peng D, et al.mBio. 2014 Dec 30;6(1):e02097-14. doi: 10.1128/mBio.02097-14.mBio. 2014.PMID:25550322Free PMC article.
- Intron targeting-mediated and endogenous gene integrity-maintaining knockin in zebrafish using the CRISPR/Cas9 system.Li J, Zhang BB, Ren YG, Gu SY, Xiang YH, Du JL.Li J, et al.Cell Res. 2015 May;25(5):634-7. doi: 10.1038/cr.2015.43. Epub 2015 Apr 7.Cell Res. 2015.PMID:25849248Free PMC article.No abstract available.
- Induced Pluripotent Stem Cells Meet Genome Editing.Hockemeyer D, Jaenisch R.Hockemeyer D, et al.Cell Stem Cell. 2016 May 5;18(5):573-86. doi: 10.1016/j.stem.2016.04.013.Cell Stem Cell. 2016.PMID:27152442Free PMC article.Review.
- Rosa26-targeted sheep gene knock-in via CRISPR-Cas9 system.Wu M, Wei C, Lian Z, Liu R, Zhu C, Wang H, Cao J, Shen Y, Zhao F, Zhang L, Mu Z, Wang Y, Wang X, Du L, Wang C.Wu M, et al.Sci Rep. 2016 Apr 11;6:24360. doi: 10.1038/srep24360.Sci Rep. 2016.PMID:27063570Free PMC article.
- Genome editing with CRISPR-Cas nucleases, base editors, transposases and prime editors.Anzalone AV, Koblan LW, Liu DR.Anzalone AV, et al.Nat Biotechnol. 2020 Jul;38(7):824-844. doi: 10.1038/s41587-020-0561-9. Epub 2020 Jun 22.Nat Biotechnol. 2020.PMID:32572269Review.
References
Publication types
MeSH terms
Substances
Related information
LinkOut - more resources
Full Text Sources
Other Literature Sources
Molecular Biology Databases
Research Materials