5-HT(2B) antagonism arrests non-canonical TGF-β1-induced valvular myofibroblast differentiation
- PMID:22940605
- PMCID: PMC3472096
- DOI: 10.1016/j.yjmcc.2012.08.012
5-HT(2B) antagonism arrests non-canonical TGF-β1-induced valvular myofibroblast differentiation
Abstract
Transforming growth factor-β1 (TGF-β1) induces myofibroblast activation of quiescent aortic valve interstitial cells (AVICs), a differentiation process implicated in calcific aortic valve disease (CAVD). The ubiquity of TGF-β1 signaling makes it difficult to target in a tissue specific manner; however, the serotonin 2B receptor (5-HT(2B)) is highly localized to cardiopulmonary tissues and agonism of this receptor displays pro-fibrotic effects in a TGF-β1-dependent manner. Therefore, we hypothesized that antagonism of 5-HT(2B) opposes TGF-β1-induced pathologic differentiation of AVICs and may offer a druggable target to prevent CAVD. To test this hypothesis, we assessed the interaction of 5-HT(2B) antagonism with canonical and non-canonical TGF-β1 pathways to inhibit TGF-β1-induced activation of isolated porcine AVICs in vitro. Here we show that AVIC activation and subsequent calcific nodule formation is completely mitigated by 5-HT(2B) antagonism. Interestingly, 5-HT(2B) antagonism does not inhibit canonical TGF-β1 signaling as identified by Smad3 phosphorylation and activation of a partial plasminogen activator inhibitor-1 promoter (PAI-1, a transcriptional target of Smad3), but prevents non-canonical p38 MAPK phosphorylation. It was initially suspected that 5-HT(2B) antagonism prevents Src tyrosine kinase phosphorylation; however, we found that this is not the case and time-lapse microscopy indicates that 5-HT(2B) antagonism prevents non-canonical TGF-β1 signaling by physically arresting Src tyrosine kinase. This study demonstrates the necessity of non-canonical TGF-β1 signaling in leading to pathologic AVIC differentiation. Moreover, we believe that the results of this study suggest 5-HT(2B) antagonism as a novel therapeutic approach for CAVD that merits further investigation.
Copyright © 2012 Elsevier Ltd. All rights reserved.
Figures
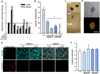
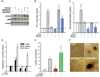
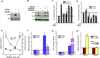
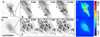
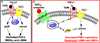
Similar articles
- 5-HT2 and 5-HT2B antagonists attenuate pro-fibrotic phenotype in human adult dermal fibroblasts by blocking TGF-β1 induced non-canonical signaling pathways including STAT3 : implications for fibrotic diseases like scleroderma.Chaturvedi S, Misra DP, Prasad N, Rastogi K, Singh H, Rai MK, Agarwal V.Chaturvedi S, et al.Int J Rheum Dis. 2018 Dec;21(12):2128-2138. doi: 10.1111/1756-185X.13386. Epub 2018 Sep 12.Int J Rheum Dis. 2018.PMID:30207074
- Cadherin-11 regulates cell-cell tension necessary for calcific nodule formation by valvular myofibroblasts.Hutcheson JD, Chen J, Sewell-Loftin MK, Ryzhova LM, Fisher CI, Su YR, Merryman WD.Hutcheson JD, et al.Arterioscler Thromb Vasc Biol. 2013 Jan;33(1):114-20. doi: 10.1161/ATVBAHA.112.300278. Epub 2012 Nov 15.Arterioscler Thromb Vasc Biol. 2013.PMID:23162011Free PMC article.
- Transforming growth factor-β1 promotes fibrosis but attenuates calcification of valvular tissue applied as a three-dimensional calcific aortic valve disease model.Jenke A, Kistner J, Saradar S, Chekhoeva A, Yazdanyar M, Bergmann AK, Rötepohl MV, Lichtenberg A, Akhyari P.Jenke A, et al.Am J Physiol Heart Circ Physiol. 2020 Nov 1;319(5):H1123-H1141. doi: 10.1152/ajpheart.00651.2019. Epub 2020 Sep 28.Am J Physiol Heart Circ Physiol. 2020.PMID:32986963
- Stimulating healthy tissue regeneration by targeting the 5-HT₂B receptor in chronic liver disease.Ebrahimkhani MR, Oakley F, Murphy LB, Mann J, Moles A, Perugorria MJ, Ellis E, Lakey AF, Burt AD, Douglass A, Wright MC, White SA, Jaffré F, Maroteaux L, Mann DA.Ebrahimkhani MR, et al.Nat Med. 2011 Nov 27;17(12):1668-73. doi: 10.1038/nm.2490.Nat Med. 2011.PMID:22120177Free PMC article.
- Integration of non-SMAD and SMAD signaling in TGF-beta1-induced plasminogen activator inhibitor type-1 gene expression in vascular smooth muscle cells.Samarakoon R, Higgins PJ.Samarakoon R, et al.Thromb Haemost. 2008 Dec;100(6):976-83.Thromb Haemost. 2008.PMID:19132220Free PMC article.Review.
Cited by
- Serotonin receptor type 2B activation augments TNF-α-induced matrix mineralization in murine valvular interstitial cells.Fong F, Xian J, Demer LL, Tintut Y.Fong F, et al.J Cell Biochem. 2021 Feb;122(2):249-258. doi: 10.1002/jcb.29847. Epub 2020 Sep 9.J Cell Biochem. 2021.PMID:32901992Free PMC article.
- Mechanobiology of myofibroblast adhesion in fibrotic cardiac disease.Schroer AK, Merryman WD.Schroer AK, et al.J Cell Sci. 2015 May 15;128(10):1865-75. doi: 10.1242/jcs.162891. Epub 2015 Apr 27.J Cell Sci. 2015.PMID:25918124Free PMC article.Review.
- Celecoxib Is Associated With Dystrophic Calcification and Aortic Valve Stenosis.Bowler MA, Raddatz MA, Johnson CL, Lindman BR, Merryman WD.Bowler MA, et al.JACC Basic Transl Sci. 2019 Feb 22;4(2):135-143. doi: 10.1016/j.jacbts.2018.12.003. eCollection 2019 Apr.JACC Basic Transl Sci. 2019.PMID:31061914Free PMC article.Review.
- Dexfenfluramine and Pergolide Cause Heart Valve Disease via Valve Metabolic Reprogramming and Ongoing Matrix Remodeling.Oury C, Maréchal P, Donis N, Hulin A, Hego A, Tridetti J, Nguyen ML, Dulgheru R, Fillet M, Nchimi A, Lancellotti P.Oury C, et al.Int J Mol Sci. 2020 Jun 3;21(11):4003. doi: 10.3390/ijms21114003.Int J Mol Sci. 2020.PMID:32503311Free PMC article.
- TGF-β mediates early angiogenesis and latent fibrosis in an Emilin1-deficient mouse model of aortic valve disease.Munjal C, Opoka AM, Osinska H, James JF, Bressan GM, Hinton RB.Munjal C, et al.Dis Model Mech. 2014 Aug;7(8):987-96. doi: 10.1242/dmm.015255.Dis Model Mech. 2014.PMID:25056700Free PMC article.
References
- Walker GA, et al. Valvular myofibroblast activation by transforming growth factor-beta: implications for pathological extracellular matrix remodeling in heart valve disease. Circ Res. 2004;95(3):253–260. - PubMed
- Bertipaglia B, et al. Cell characterization of porcine aortic valve and decellularized leaflets repopulated with aortic valve interstitial cells: the VESALIO Project (Vitalitate Exornatum Succedaneum Aorticum Labore Ingenioso Obtenibitur) Ann Thorac Surg. 2003;75(4):1274–1282. - PubMed
- Akat K, Borggrefe M, Kaden JJ. Aortic valve calcification: basic science to clinical practice. Heart. 2009;95(8):616–623. - PubMed
- Jian B, et al. Progression of aortic valve stenosis: TGF-beta1 is present in calcified aortic valve cusps and promotes aortic valve interstitial cell calcification via apoptosis. Ann Thorac Surg. 2003;75(2):457–465. discussion 465-6. - PubMed
Publication types
MeSH terms
Substances
Related information
Grants and funding
LinkOut - more resources
Full Text Sources
Miscellaneous