Function of Wnt/β-catenin in counteracting Tcf3 repression through the Tcf3-β-catenin interaction
- PMID:22573616
- PMCID: PMC3357906
- DOI: 10.1242/dev.076067
Function of Wnt/β-catenin in counteracting Tcf3 repression through the Tcf3-β-catenin interaction
Abstract
The canonical Wnt/β-catenin signaling pathway classically functions through the activation of target genes by Tcf/Lef-β-catenin complexes. In contrast to β-catenin-dependent functions described for Tcf1, Tcf4 and Lef1, the known embryonic functions for Tcf3 in mice, frogs and fish are consistent with β-catenin-independent repressor activity. In this study, we genetically define Tcf3-β-catenin functions in mice by generating a Tcf3ΔN knock-in mutation that specifically ablates Tcf3-β-catenin. Mouse embryos homozygous for the knock-in mutation (Tcf3(ΔN/ΔN)) progress through gastrulation without apparent defects, thus genetically proving that Tcf3 function during gastrulation is independent of β-catenin interaction. Tcf3(ΔN/ΔN) mice were not viable, and several post-gastrulation defects revealed the first in vivo functions of Tcf3-β-catenin interaction affecting limb development, vascular integrity, neural tube closure and eyelid closure. Interestingly, the etiology of defects indicated an indirect role for Tcf3-β-catenin in the activation of target genes. Tcf3 directly represses transcription of Lef1, which is stimulated by Wnt/β-catenin activity. These genetic data indicate that Tcf3-β-catenin is not necessary to activate target genes directly. Instead, our findings support the existence of a regulatory circuit whereby Wnt/β-catenin counteracts Tcf3 repression of Lef1, which subsequently activates target gene expression via Lef1-β-catenin complexes. We propose that the Tcf/Lef circuit model provides a mechanism downstream of β-catenin stability for controlling the strength of Wnt signaling activity during embryonic development.
Figures
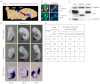
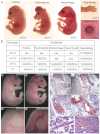
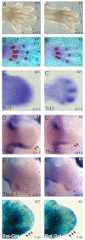
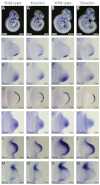
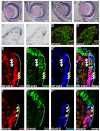
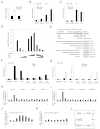
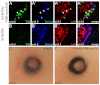
Similar articles
- Tcf3 represses Wnt-β-catenin signaling and maintains neural stem cell population during neocortical development.Kuwahara A, Sakai H, Xu Y, Itoh Y, Hirabayashi Y, Gotoh Y.Kuwahara A, et al.PLoS One. 2014 May 15;9(5):e94408. doi: 10.1371/journal.pone.0094408. eCollection 2014.PLoS One. 2014.PMID:24832538Free PMC article.
- A dynamic exchange of TCF3 and TCF4 transcription factors controls MYC expression in colorectal cancer cells.Shah M, Rennoll SA, Raup-Konsavage WM, Yochum GS.Shah M, et al.Cell Cycle. 2015;14(3):323-32. doi: 10.4161/15384101.2014.980643.Cell Cycle. 2015.PMID:25659031Free PMC article.
- Mouse Tcf3 represses canonical Wnt signaling by either competing for β-catenin binding or through occupation of DNA-binding sites.Solberg N, Machon O, Machonova O, Krauss S.Solberg N, et al.Mol Cell Biochem. 2012 Jun;365(1-2):53-63. doi: 10.1007/s11010-012-1243-9. Epub 2012 Jan 22.Mol Cell Biochem. 2012.PMID:22270545
- Wnt signalling in mouse gastrulation and anterior development: new players in the pathway and signal output.Arkell RM, Fossat N, Tam PP.Arkell RM, et al.Curr Opin Genet Dev. 2013 Aug;23(4):454-60. doi: 10.1016/j.gde.2013.03.001. Epub 2013 Apr 19.Curr Opin Genet Dev. 2013.PMID:23608663Review.
- TCF: Lady Justice casting the final verdict on the outcome of Wnt signalling.Brantjes H, Barker N, van Es J, Clevers H.Brantjes H, et al.Biol Chem. 2002 Feb;383(2):255-61. doi: 10.1515/BC.2002.027.Biol Chem. 2002.PMID:11934263Review.
Cited by
- Tcf7l1 prepares epiblast cells in the gastrulating mouse embryo for lineage specification.Hoffman JA, Wu CI, Merrill BJ.Hoffman JA, et al.Development. 2013 Apr;140(8):1665-75. doi: 10.1242/dev.087387. Epub 2013 Mar 13.Development. 2013.PMID:23487311Free PMC article.
- Repression of MAP3K1 expression and JNK activity by canonical Wnt signaling.Meng Q, Mongan M, Wang J, Xia Y.Meng Q, et al.Dev Biol. 2018 Aug 15;440(2):129-136. doi: 10.1016/j.ydbio.2018.05.008. Epub 2018 May 19.Dev Biol. 2018.PMID:29787744Free PMC article.
- miR-218 directs a Wnt signaling circuit to promote differentiation of osteoblasts and osteomimicry of metastatic cancer cells.Hassan MQ, Maeda Y, Taipaleenmaki H, Zhang W, Jafferji M, Gordon JA, Li Z, Croce CM, van Wijnen AJ, Stein JL, Stein GS, Lian JB.Hassan MQ, et al.J Biol Chem. 2012 Dec 7;287(50):42084-92. doi: 10.1074/jbc.M112.377515. Epub 2012 Oct 11.J Biol Chem. 2012.PMID:23060446Free PMC article.
- Mest attenuates CCl4-induced liver fibrosis in rats by inhibiting the Wnt/β-catenin signaling pathway.Li W, Zhu C, Li Y, Wu Q, Gao R.Li W, et al.Gut Liver. 2014 May;8(3):282-91. doi: 10.5009/gnl.2014.8.3.282.Gut Liver. 2014.PMID:24827625Free PMC article.
- Wnt signaling regulates the lineage differentiation potential of mouse embryonic stem cells through Tcf3 down-regulation.Atlasi Y, Noori R, Gaspar C, Franken P, Sacchetti A, Rafati H, Mahmoudi T, Decraene C, Calin GA, Merrill BJ, Fodde R.Atlasi Y, et al.PLoS Genet. 2013 May;9(5):e1003424. doi: 10.1371/journal.pgen.1003424. Epub 2013 May 2.PLoS Genet. 2013.PMID:23658527Free PMC article.
References
- Arnold S. J., Stappert J., Bauer A., Kispert A., Herrmann B. G., Kemler R. (2000). Brachyury is a target gene of the Wnt/beta-catenin signaling pathway. Mech. Dev. 91, 249–258 - PubMed
- Behrens J., von Kries J. P., Kuhl M., Bruhn L., Wedlich D., Grosschedl R., Birchmeier W. (1996). Functional interaction of beta-catenin with the transcription factor LEF-1. Nature 382, 638–642 - PubMed
Publication types
MeSH terms
Substances
Related information
Grants and funding
LinkOut - more resources
Full Text Sources
Molecular Biology Databases