Gelsolin amyloidosis: genetics, biochemistry, pathology and possible strategies for therapeutic intervention
- PMID:22360545
- PMCID: PMC3337338
- DOI: 10.3109/10409238.2012.661401
Gelsolin amyloidosis: genetics, biochemistry, pathology and possible strategies for therapeutic intervention
Abstract
Protein misassembly into aggregate structures, including cross-β-sheet amyloid fibrils, is linked to diseases characterized by the degeneration of post-mitotic tissue. While amyloid fibril deposition in the extracellular space certainly disrupts cellular and tissue architecture late in the course of amyloid diseases, strong genetic, pathological and pharmacologic evidence suggests that the process of amyloid fibril formation itself, known as amyloidogenesis, likely causes these maladies. It seems that the formation of oligomeric aggregates during the amyloidogenesis process causes the proteotoxicity and cytotoxicity characteristic of these disorders. Herein, we review what is known about the genetics, biochemistry and pathology of familial amyloidosis of Finnish type (FAF) or gelsolin amyloidosis. Briefly, autosomal dominant D187N or D187Y mutations compromise Ca(2+) binding in domain 2 of gelsolin, allowing domain 2 to sample unfolded conformations. When domain 2 is unfolded, gelsolin is subject to aberrant furin endoproteolysis as it passes through the Golgi on its way to the extracellular space. The resulting C-terminal 68 kDa fragment (C68) is susceptible to extracellular endoproteolytic events, possibly mediated by a matrix metalloprotease, affording 8 and 5 kDa amyloidogenic fragments of gelsolin. These amyloidogenic fragments deposit systemically, causing a variety of symptoms including corneal lattice dystrophy and neurodegeneration. The first murine model of the disease recapitulates the aberrant processing of mutant plasma gelsolin, amyloid deposition, and the degenerative phenotype. We use what we have learned from our biochemical studies, as well as insight from mouse and human pathology to propose therapeutic strategies that may halt the progression of FAF.
Figures
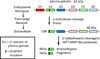
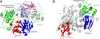
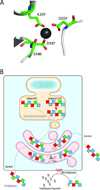
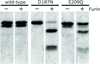
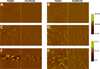
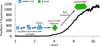
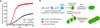
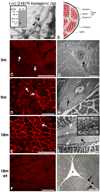
Similar articles
- The 8 and 5 kDa fragments of plasma gelsolin form amyloid fibrils by a nucleated polymerization mechanism, while the 68 kDa fragment is not amyloidogenic.Solomon JP, Yonemoto IT, Murray AN, Price JL, Powers ET, Balch WE, Kelly JW.Solomon JP, et al.Biochemistry. 2009 Dec 8;48(48):11370-80. doi: 10.1021/bi901368e.Biochemistry. 2009.PMID:19904968Free PMC article.
- Nanobody interaction unveils structure, dynamics and proteotoxicity of the Finnish-type amyloidogenic gelsolin variant.Giorgino T, Mattioni D, Hassan A, Milani M, Mastrangelo E, Barbiroli A, Verhelle A, Gettemans J, Barzago MM, Diomede L, de Rosa M.Giorgino T, et al.Biochim Biophys Acta Mol Basis Dis. 2019 Mar 1;1865(3):648-660. doi: 10.1016/j.bbadis.2019.01.010. Epub 2019 Jan 6.Biochim Biophys Acta Mol Basis Dis. 2019.PMID:30625383
- Gelsolin domain 2 Ca2+ affinity determines susceptibility to furin proteolysis and familial amyloidosis of finnish type.Huff ME, Page LJ, Balch WE, Kelly JW.Huff ME, et al.J Mol Biol. 2003 Nov 14;334(1):119-27. doi: 10.1016/j.jmb.2003.09.029.J Mol Biol. 2003.PMID:14596804
- Ca2+ binding protects against gelsolin amyloidosis.Page LJ, Huff ME, Kelly JW, Balch WE.Page LJ, et al.Biochem Biophys Res Commun. 2004 Oct 1;322(4):1105-10. doi: 10.1016/j.bbrc.2004.07.125.Biochem Biophys Res Commun. 2004.PMID:15336957Review.
- [Hereditary gelsolin amyloidosis--40 years of Meretoja disease].Kiuru-Enari S, Haltia M.Kiuru-Enari S, et al.Duodecim. 2010;126(10):1162-71.Duodecim. 2010.PMID:20597346Review.Finnish.
Cited by
- Ocular surface development and gene expression.Swamynathan SK.Swamynathan SK.J Ophthalmol. 2013;2013:103947. doi: 10.1155/2013/103947. Epub 2013 Feb 21.J Ophthalmol. 2013.PMID:23533700Free PMC article.
- Gelsolin Amyloidogenesis Is Effectively Modulated by Curcumin and Emetine Conjugated PLGA Nanoparticles.Srivastava A, Arya P, Goel S, Kundu B, Mishra P, Fnu A.Srivastava A, et al.PLoS One. 2015 May 21;10(5):e0127011. doi: 10.1371/journal.pone.0127011. eCollection 2015.PLoS One. 2015.PMID:25996685Free PMC article.
- Actin and Actin-Binding Proteins.Pollard TD.Pollard TD.Cold Spring Harb Perspect Biol. 2016 Aug 1;8(8):a018226. doi: 10.1101/cshperspect.a018226.Cold Spring Harb Perspect Biol. 2016.PMID:26988969Free PMC article.Review.
- Interplay between Oxidative Stress, Inflammation, and Amyloidosis in the Anterior Segment of the Eye; Its Pathological Implications.Perez-Garmendia R, Lopez de Eguileta Rodriguez A, Ramos-Martinez I, Zuñiga NM, Gonzalez-Salinas R, Quiroz-Mercado H, Zenteno E, Hernández ER, Hernández-Zimbrón LF.Perez-Garmendia R, et al.Oxid Med Cell Longev. 2020 Jun 3;2020:6286105. doi: 10.1155/2020/6286105. eCollection 2020.Oxid Med Cell Longev. 2020.PMID:32566091Free PMC article.Review.
- Ocular Involvement in Hereditary Amyloidosis.Minnella AM, Rissotto R, Antoniazzi E, Di Girolamo M, Luigetti M, Maceroni M, Bacherini D, Falsini B, Rizzo S, Obici L.Minnella AM, et al.Genes (Basel). 2021 Jun 22;12(7):955. doi: 10.3390/genes12070955.Genes (Basel). 2021.PMID:34206500Free PMC article.Review.
References
- Ardalan MR, Shoja MM, Kiuru-Enari S. Amyloidosis-related nephrotic syndrome due to a G654A gelsolin mutation: the first report from the Middle East. Nephrol Dial Transplant. 2007;22:272–275. - PubMed
- Arumugam TV, Cheng Y-L, Choi Y, Choi Y-H, Yang S, Yun Y-K, Park J-S, Yang DK, Thundyil J, Gelderblom M, Karamyan VT, Tang S-C, Chan SL, Magnus T, Sobey CG, Jo D-G. Evidence that γ-secretase-mediated Notch signaling induces neuronal cell death via the nuclear factor-κB-Bcl-2-interacting mediator of cell death pathway in ischemic stroke. Mol Pharmacol. 2011;80:23–31. - PubMed
- Ashish, Paine MS, Perryman PB, Yang L, Yin HL, Krueger JK. Global structure changes associated with Ca2+ activation of full-length human plasma gelsolin. J Biol Chem. 2007;282:25884–25892. - PubMed
- Balch WE, Morimoto RI, Dillin A, Kelly JW. Adapting proteostasis for disease intervention. Science. 2008;319:916–919. - PubMed
- Baures PW, Oza VB, Peterson SA, Kelly JW. Synthesis and evaluation of inhibitors of transthyretin amyloid formation based on the nonsteroidal antiinflammatory drug flufenamic acid. Bioorg Med Chem. 1999;7:1339–1347. - PubMed
Publication types
MeSH terms
Substances
Supplementary concepts
Related information
Grants and funding
LinkOut - more resources
Full Text Sources
Other Literature Sources
Research Materials
Miscellaneous