Astrocytes mediate in vivo cholinergic-induced synaptic plasticity
- PMID:22347811
- PMCID: PMC3279365
- DOI: 10.1371/journal.pbio.1001259
Astrocytes mediate in vivo cholinergic-induced synaptic plasticity
Abstract
Long-term potentiation (LTP) of synaptic transmission represents the cellular basis of learning and memory. Astrocytes have been shown to regulate synaptic transmission and plasticity. However, their involvement in specific physiological processes that induce LTP in vivo remains unknown. Here we show that in vivo cholinergic activity evoked by sensory stimulation or electrical stimulation of the septal nucleus increases Ca²⁺ in hippocampal astrocytes and induces LTP of CA3-CA1 synapses, which requires cholinergic muscarinic (mAChR) and metabotropic glutamate receptor (mGluR) activation. Stimulation of cholinergic pathways in hippocampal slices evokes astrocyte Ca²⁺ elevations, postsynaptic depolarizations of CA1 pyramidal neurons, and LTP of transmitter release at single CA3-CA1 synapses. Like in vivo, these effects are mediated by mAChRs, and this cholinergic-induced LTP (c-LTP) also involves mGluR activation. Astrocyte Ca²⁺ elevations and LTP are absent in IP₃R2 knock-out mice. Downregulating astrocyte Ca²⁺ signal by loading astrocytes with BAPTA or GDPβS also prevents LTP, which is restored by simultaneous astrocyte Ca²⁺ uncaging and postsynaptic depolarization. Therefore, cholinergic-induced LTP requires astrocyte Ca²⁺ elevations, which stimulate astrocyte glutamate release that activates mGluRs. The cholinergic-induced LTP results from the temporal coincidence of the postsynaptic activity and the astrocyte Ca²⁺ signal simultaneously evoked by cholinergic activity. Therefore, the astrocyte Ca²⁺ signal is necessary for cholinergic-induced synaptic plasticity, indicating that astrocytes are directly involved in brain storage information.
Conflict of interest statement
The authors have declared that no competing interests exist.
Figures
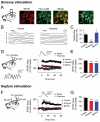
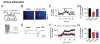
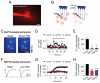
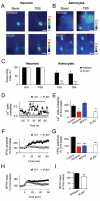
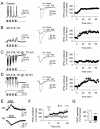
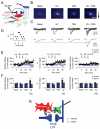
Comment in
- A new look at "filler cells" in the brain reveals their role in learning.Weaver J.Weaver J.PLoS Biol. 2012 Feb;10(2):e1001263. doi: 10.1371/journal.pbio.1001263. Epub 2012 Feb 14.PLoS Biol. 2012.PMID:22347813Free PMC article.
Similar articles
- Glutamate released spontaneously from astrocytes sets the threshold for synaptic plasticity.Bonansco C, Couve A, Perea G, Ferradas CÁ, Roncagliolo M, Fuenzalida M.Bonansco C, et al.Eur J Neurosci. 2011 Apr;33(8):1483-92. doi: 10.1111/j.1460-9568.2011.07631.x. Epub 2011 Mar 14.Eur J Neurosci. 2011.PMID:21395864
- Group I metabotropic glutamate receptors regulate the frequency-response function of hippocampal CA1 synapses for the induction of LTP and LTD.van Dam EJ, Kamal A, Artola A, de Graan PN, Gispen WH, Ramakers GM.van Dam EJ, et al.Eur J Neurosci. 2004 Jan;19(1):112-8. doi: 10.1111/j.1460-9568.2004.03103.x.Eur J Neurosci. 2004.PMID:14750969
- Astrocytes potentiate transmitter release at single hippocampal synapses.Perea G, Araque A.Perea G, et al.Science. 2007 Aug 24;317(5841):1083-6. doi: 10.1126/science.1144640.Science. 2007.PMID:17717185
- The active role of astrocytes in synaptic transmission.Vesce S, Bezzi P, Volterra A.Vesce S, et al.Cell Mol Life Sci. 1999 Dec;56(11-12):991-1000. doi: 10.1007/s000180050488.Cell Mol Life Sci. 1999.PMID:11212330Free PMC article.Review.
- Evolution of Glutamate Metabolism viaGLUD2 Enhances Lactate-Dependent Synaptic Plasticity and Complex Cognition.Plaitakis A, Sidiropoulou K, Kotzamani D, Litso I, Zaganas I, Spanaki C.Plaitakis A, et al.Int J Mol Sci. 2024 May 13;25(10):5297. doi: 10.3390/ijms25105297.Int J Mol Sci. 2024.PMID:38791334Free PMC article.Review.
Cited by
- Modeling Working Memory in a Spiking Neuron Network Accompanied by Astrocytes.Gordleeva SY, Tsybina YA, Krivonosov MI, Ivanchenko MV, Zaikin AA, Kazantsev VB, Gorban AN.Gordleeva SY, et al.Front Cell Neurosci. 2021 Mar 31;15:631485. doi: 10.3389/fncel.2021.631485. eCollection 2021.Front Cell Neurosci. 2021.PMID:33867939Free PMC article.
- Morphological plasticity of astroglia: Understanding synaptic microenvironment.Heller JP, Rusakov DA.Heller JP, et al.Glia. 2015 Dec;63(12):2133-51. doi: 10.1002/glia.22821. Epub 2015 Mar 18.Glia. 2015.PMID:25782611Free PMC article.Review.
- Neuron-glia signaling in developing retina mediated by neurotransmitter spillover.Rosa JM, Bos R, Sack GS, Fortuny C, Agarwal A, Bergles DE, Flannery JG, Feller MB.Rosa JM, et al.Elife. 2015 Aug 14;4:e09590. doi: 10.7554/eLife.09590.Elife. 2015.PMID:26274565Free PMC article.
- Genetic targeting of astrocytes to combat neurodegenerative disease.Kery R, Chen APF, Kirschen GW.Kery R, et al.Neural Regen Res. 2020 Feb;15(2):199-211. doi: 10.4103/1673-5374.265541.Neural Regen Res. 2020.PMID:31552885Free PMC article.
- Diversity and Specificity of Astrocyte-neuron Communication.Durkee CA, Araque A.Durkee CA, et al.Neuroscience. 2019 Jan 1;396:73-78. doi: 10.1016/j.neuroscience.2018.11.010. Epub 2018 Nov 17.Neuroscience. 2019.PMID:30458223Free PMC article.Review.
References
- Perea G, Navarrete M, Araque A. Tripartite synapses: astrocytes process and control synaptic information. Trends Neurosci. 2009;32:421–431. - PubMed
- Volterra A, Meldolesi J. Astrocytes, from brain glue to communication elements: the revolution continues. Nat Rev Neurosci. 2005;6:626–640. - PubMed
- Di Castro M. A, Chuquet J, Liaudet N, Bhaukaurally K, Santello M, Bouvier D, Tiret P, Volterra A. Local Ca(2+) detection and modulation of synaptic release by astrocytes. Nat Neurosci. 2011;14:1276–1284. - PubMed
Publication types
MeSH terms
Substances
Related information
LinkOut - more resources
Full Text Sources
Other Literature Sources
Molecular Biology Databases
Miscellaneous