Enthalpy-entropy compensation and cooperativity as thermodynamic epiphenomena of structural flexibility in ligand-receptor interactions
- PMID:22342886
- PMCID: PMC3349339
- DOI: 10.1016/j.jmb.2012.01.057
Enthalpy-entropy compensation and cooperativity as thermodynamic epiphenomena of structural flexibility in ligand-receptor interactions
Abstract
Ligand binding is a thermodynamically cooperative process in many biochemical systems characterized by the conformational flexibility of the reactants. However, the contribution of conformational entropy to cooperativity of ligation needs to be elucidated. Here, we perform kinetic and thermodynamic analyses on a panel of cycle-mutated peptides, derived from influenza H3 HA(306-319), interacting with wild type and a mutant HLA-DR. We observe that, within a certain range of peptide affinity, this system shows isothermal entropy-enthalpy compensation (iEEC). The incremental increases in conformational entropy measured as disruptive mutations are added in the ligand or receptor are more than sufficient in magnitude to account for the experimentally observed lack of free-energy decrease cooperativity. Beyond this affinity range, compensation is not observed, and therefore, the ability of the residual interactions to form a stable complex decreases in an exponential fashion. Taken together, our results indicate that cooperativity and iEEC constitute the thermodynamic epiphenomena of the structural fluctuation that accompanies ligand-receptor complex formation in flexible systems. Therefore, ligand binding affinity prediction needs to consider how each source of binding energy contributes synergistically to the folding and kinetic stability of the complex in a process based on the trade-off between structural tightening and restraint of conformational mobility.
Copyright © 2012 Elsevier Ltd. All rights reserved.
Figures
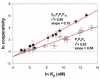
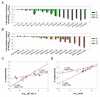
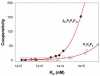
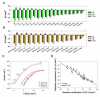
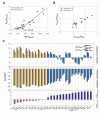
Similar articles
- Analysis of the thermodynamics of binding of an SH3 domain to proline-rich peptides using a chimeric fusion protein.Candel AM, van Nuland NA, Martin-Sierra FM, Martinez JC, Conejero-Lara F.Candel AM, et al.J Mol Biol. 2008 Mar 14;377(1):117-35. doi: 10.1016/j.jmb.2007.11.060. Epub 2007 Nov 28.J Mol Biol. 2008.PMID:18234212
- The high-resolution NMR structure of the R21A Spc-SH3:P41 complex: understanding the determinants of binding affinity by comparison with Abl-SH3.Casares S, Ab E, Eshuis H, Lopez-Mayorga O, van Nuland NA, Conejero-Lara F.Casares S, et al.BMC Struct Biol. 2007 Apr 2;7:22. doi: 10.1186/1472-6807-7-22.BMC Struct Biol. 2007.PMID:17407569Free PMC article.
- Constraining binding hot spots: NMR and molecular dynamics simulations provide a structural explanation for enthalpy-entropy compensation in SH2-ligand binding.Ward JM, Gorenstein NM, Tian J, Martin SF, Post CB.Ward JM, et al.J Am Chem Soc. 2010 Aug 18;132(32):11058-70. doi: 10.1021/ja910535j.J Am Chem Soc. 2010.PMID:20698672Free PMC article.
- Conformational dynamics and thermodynamics of protein-ligand binding studied by NMR relaxation.Akke M.Akke M.Biochem Soc Trans. 2012 Apr;40(2):419-23. doi: 10.1042/BST20110750.Biochem Soc Trans. 2012.PMID:22435823Review.
- Ligand Binding Thermodynamics in Drug Discovery: Still a Hot Tip?Geschwindner S, Ulander J, Johansson P.Geschwindner S, et al.J Med Chem. 2015 Aug 27;58(16):6321-35. doi: 10.1021/jm501511f. Epub 2015 May 11.J Med Chem. 2015.PMID:25915439Review.
Cited by
- For many but not for all: how the conformational flexibility of the peptide/MHCII complex shapes epitope selection.Ferrante A.Ferrante A.Immunol Res. 2013 May;56(1):85-95. doi: 10.1007/s12026-012-8342-2.Immunol Res. 2013.PMID:22753017Free PMC article.Review.
- Entropy Effects in Intermolecular Associations of Crown-Ethers and Cyclodextrins with Amino Acids in Aqueous and in Non-Aqueous Media.Usacheva T, Terekhova I, Alister D, Agafonov M, Kuranova N, Tyurin D, Sharnin V.Usacheva T, et al.Entropy (Basel). 2021 Dec 24;24(1):24. doi: 10.3390/e24010024.Entropy (Basel). 2021.PMID:35052050Free PMC article.
- Configurational space discretization and free energy calculation in complex molecular systems.Wang K, Long S, Tian P.Wang K, et al.Sci Rep. 2016 Mar 14;6:22217. doi: 10.1038/srep22217.Sci Rep. 2016.PMID:26974524Free PMC article.
- Assembly path dependence of telomeric DNA compaction by TRF1, TIN2, and SA1.Liu M, Pan H, Kaur P, Wang LJ, Jin M, Detwiler AC, Opresko PL, Tao YJ, Wang H, Riehn R.Liu M, et al.Biophys J. 2023 May 16;122(10):1822-1832. doi: 10.1016/j.bpj.2023.04.014. Epub 2023 Apr 20.Biophys J. 2023.PMID:37081787Free PMC article.
- Entropy-enthalpy Compensation of Biomolecular Systems in Aqueous Phase: a Dry Perspective.Movileanu L, Schiff EA.Movileanu L, et al.Monatsh Chem. 2013 Jan 1;144(1):59-65. doi: 10.1007/s00706-012-0839-9.Monatsh Chem. 2013.PMID:23976794Free PMC article.
References
- Li Z, Lazaridis T. Water at biomolecular binding interfaces. Phys Chem Chem Phys. 2007;9:573–81. - PubMed
- Fischer E. Einfluss der Configuration auf die Wirkung der Enzyme. Ber Dtsch Chem Ges. 1894;27:2984–2993.
- Lumry R. Uses of enthalpy-entropy compensation in protein research. Biophys Chem. 2003;105:545–57. - PubMed
Publication types
MeSH terms
Substances
Related information
Grants and funding
LinkOut - more resources
Full Text Sources
Research Materials