Cortical correlates of fitts' law
- PMID:22275888
- PMCID: PMC3250970
- DOI: 10.3389/fnint.2011.00085
Cortical correlates of fitts' law
Abstract
Fitts' law describes the fundamental trade-off between movement accuracy and speed: it states that the duration of reaching movements is a function of target size (TS) and distance. While Fitts' law has been extensively studied in ergonomics and has guided the design of human-computer interfaces, there have been few studies on its neuronal correlates. To elucidate sensorimotor cortical activity underlying Fitts' law, we implanted two monkeys with multielectrode arrays in the primary motor (M1) and primary somatosensory (S1) cortices. The monkeys performed reaches with a joystick-controlled cursor toward targets of different size. The reaction time (RT), movement time, and movement velocity changed with TS, and M1 and S1 activity reflected these changes. Moreover, modifications of cortical activity could not be explained by changes of movement parameters alone, but required TS as an additional parameter. Neuronal representation of TS was especially prominent during the early RT period where it influenced the slope of the firing rate rise preceding movement initiation. During the movement period, cortical activity was correlated with movement velocity. Neural decoders were applied to simultaneously decode TS and motor parameters from cortical modulations. We suggest that sensorimotor cortex activity reflects the characteristics of both the movement and the target. Classifiers that extract these parameters from cortical ensembles could improve neuroprosthetic control.
Keywords: Fitts’ law; brain–machine interface; decision making; motor cortex; neurophysiology; neuroprosthetics; sensorimotor transformation; somatosensory cortex.
Figures
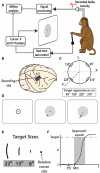
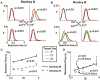
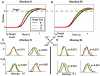
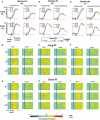
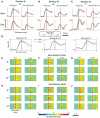
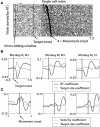
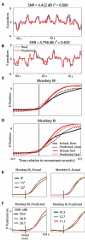
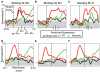
Similar articles
- Hemodynamic activity is not parsimoniously tuned to index-of-difficulty in movement with dual requirements on speed-accuracy.Ji H, Chen Z, Qiao Y, Yan J, Chen G, Luo Q, Cui L, Zong Y, Xie Q, Niu CM.Ji H, et al.Front Hum Neurosci. 2024 Jul 9;18:1398601. doi: 10.3389/fnhum.2024.1398601. eCollection 2024.Front Hum Neurosci. 2024.PMID:39045507Free PMC article.
- Signal-independent noise in intracortical brain-computer interfaces causes movement time properties inconsistent with Fitts' law.Willett FR, Murphy BA, Memberg WD, Blabe CH, Pandarinath C, Walter BL, Sweet JA, Miller JP, Henderson JM, Shenoy KV, Hochberg LR, Kirsch RF, Ajiboye AB.Willett FR, et al.J Neural Eng. 2017 Apr;14(2):026010. doi: 10.1088/1741-2552/aa5990. Epub 2017 Feb 8.J Neural Eng. 2017.PMID:28177925Free PMC article.
- Estimation of psychomotor delay from the Fitts' law coefficients.Beamish D, Bhatti S, Chubbs CS, MacKenzie IS, Wu J, Jing Z.Beamish D, et al.Biol Cybern. 2009 Oct;101(4):279-96. doi: 10.1007/s00422-009-0336-3. Epub 2009 Oct 28.Biol Cybern. 2009.PMID:19862551
- Predictive feedback control and Fitts' law.Gawthrop P, Lakie M, Loram I.Gawthrop P, et al.Biol Cybern. 2008 Mar;98(3):229-38. doi: 10.1007/s00422-007-0206-9. Epub 2008 Jan 5.Biol Cybern. 2008.PMID:18180947
- Multielectrode Recordings in the Somatosensory System.Wiest M, Thomson E, Meloy J.Wiest M, et al.In: Nicolelis MAL, editor. Methods for Neural Ensemble Recordings. 2nd edition. Boca Raton (FL): CRC Press/Taylor & Francis; 2008. Chapter 6.In: Nicolelis MAL, editor. Methods for Neural Ensemble Recordings. 2nd edition. Boca Raton (FL): CRC Press/Taylor & Francis; 2008. Chapter 6.PMID:21204443Free Books & Documents.Review.
Cited by
- Evaluation of speed-accuracy trade-off in a computer task in individuals with cerebral palsy: a cross-sectional study.Fernani DCGL, Prado MTA, da Silva TD, Massetti T, de Abreu LC, Magalhães FH, Dawes H, de Mello Monteiro CB.Fernani DCGL, et al.BMC Neurol. 2017 Jul 27;17(1):143. doi: 10.1186/s12883-017-0920-4.BMC Neurol. 2017.PMID:28750603Free PMC article.Clinical Trial.
- Stopping is not an option: the evolution of unstoppable motion elements (primitives).Sosnik R, Chaim E, Flash T.Sosnik R, et al.J Neurophysiol. 2015 Aug;114(2):846-56. doi: 10.1152/jn.00341.2015. Epub 2015 Jun 3.J Neurophysiol. 2015.PMID:26041827Free PMC article.
- Chronic, wireless recordings of large-scale brain activity in freely moving rhesus monkeys.Schwarz DA, Lebedev MA, Hanson TL, Dimitrov DF, Lehew G, Meloy J, Rajangam S, Subramanian V, Ifft PJ, Li Z, Ramakrishnan A, Tate A, Zhuang KZ, Nicolelis MA.Schwarz DA, et al.Nat Methods. 2014 Jun;11(6):670-6. doi: 10.1038/nmeth.2936. Epub 2014 Apr 28.Nat Methods. 2014.PMID:24776634Free PMC article.
- Advancing brain-machine interfaces: moving beyond linear state space models.Rouse AG, Schieber MH.Rouse AG, et al.Front Syst Neurosci. 2015 Jul 28;9:108. doi: 10.3389/fnsys.2015.00108. eCollection 2015.Front Syst Neurosci. 2015.PMID:26283932Free PMC article.
- Reprogramming movements: extraction of motor intentions from cortical ensemble activity when movement goals change.Ifft PJ, Lebedev MA, Nicolelis MA.Ifft PJ, et al.Front Neuroeng. 2012 Jul 18;5:16. doi: 10.3389/fneng.2012.00016. eCollection 2012.Front Neuroeng. 2012.PMID:22826698Free PMC article.
References
- Alexander G. E., Crutcher M. D. (1990). Neural representations of the target (goal) of visually guided arm movements in three motor areas of the monkey. J. Neurophysiol. 64, 164–178 - PubMed
- Bohan M., Longstaff M. G., Van Gemmert A. W., Rand M. K., Stelmach G. E. (2003). Effects of target height and width on 2D pointing movement duration and kinematics. Motor Control 7, 278–289 - PubMed
Related information
Grants and funding
LinkOut - more resources
Full Text Sources