Activation of IFN-β expression by a viral mRNA through RNase L and MDA5
- PMID:21245317
- PMCID: PMC3033319
- DOI: 10.1073/pnas.1012409108
Activation of IFN-β expression by a viral mRNA through RNase L and MDA5
Abstract
IFNs play a critical role in innate immunity against viral infections. Melanoma differentiation-associated protein 5 (MDA5), an RNA helicase, is a key component in activating the expression of type I IFNs in response to certain types of viral infection. MDA5 senses noncellular RNA and triggers the signaling cascade that leads to IFN production. Synthetic double-stranded RNAs are known activators of MDA5. Natural single-stranded RNAs have not been reported to activate MDA5, however. We have serendipitously identified a viral mRNA from parainfluenza virus 5 (PIV5) that activates IFN expression through MDA5. We provide evidence that the signaling pathway includes the antiviral enzyme RNase L. The L mRNA of PIV5 activated expression of IFN-β. We have mapped the RNA to a region of 430 nucleotides within the L mRNA of PIV5. Our results indicate that a viral mRNA, with 5'-cap and 3'-poly (A), can activate IFN expression through an RNase L-MDA5 pathway.
Conflict of interest statement
The authors declare no conflict of interest.
Figures
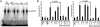
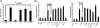
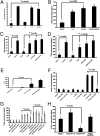
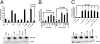
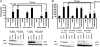
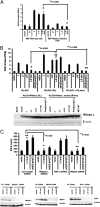
Similar articles
- RNase L Amplifies Interferon Signaling by Inducing Protein Kinase R-Mediated Antiviral Stress Granules.Manivannan P, Siddiqui MA, Malathi K.Manivannan P, et al.J Virol. 2020 Jun 16;94(13):e00205-20. doi: 10.1128/JVI.00205-20. Print 2020 Jun 16.J Virol. 2020.PMID:32295917Free PMC article.
- Small self-RNA generated by RNase L amplifies antiviral innate immunity.Malathi K, Dong B, Gale M Jr, Silverman RH.Malathi K, et al.Nature. 2007 Aug 16;448(7155):816-9. doi: 10.1038/nature06042. Epub 2007 Jul 25.Nature. 2007.PMID:17653195Free PMC article.
- Interferon-Inducible Oligoadenylate Synthetase-Like Protein Acts as an Antiviral Effector against Classical Swine Fever Virus via the MDA5-Mediated Type I Interferon-Signaling Pathway.Li LF, Yu J, Zhang Y, Yang Q, Li Y, Zhang L, Wang J, Li S, Luo Y, Sun Y, Qiu HJ.Li LF, et al.J Virol. 2017 May 12;91(11):e01514-16. doi: 10.1128/JVI.01514-16. Print 2017 Jun 1.J Virol. 2017.PMID:28331099Free PMC article.
- MDA5-filament, dynamics and disease.del Toro Duany Y, Wu B, Hur S.del Toro Duany Y, et al.Curr Opin Virol. 2015 Jun;12:20-5. doi: 10.1016/j.coviro.2015.01.011. Epub 2015 Feb 9.Curr Opin Virol. 2015.PMID:25676875Free PMC article.Review.
- Effects of type 1 diabetes-associated IFIH1 polymorphisms on MDA5 function and expression.Looney BM, Xia CQ, Concannon P, Ostrov DA, Clare-Salzler MJ.Looney BM, et al.Curr Diab Rep. 2015 Nov;15(11):96. doi: 10.1007/s11892-015-0656-8.Curr Diab Rep. 2015.PMID:26385483Review.
Cited by
- A Conserved Histidine in the RNA Sensor RIG-I Controls Immune Tolerance to N1-2'O-Methylated Self RNA.Schuberth-Wagner C, Ludwig J, Bruder AK, Herzner AM, Zillinger T, Goldeck M, Schmidt T, Schmid-Burgk JL, Kerber R, Wolter S, Stümpel JP, Roth A, Bartok E, Drosten C, Coch C, Hornung V, Barchet W, Kümmerer BM, Hartmann G, Schlee M.Schuberth-Wagner C, et al.Immunity. 2015 Jul 21;43(1):41-51. doi: 10.1016/j.immuni.2015.06.015. Epub 2015 Jul 14.Immunity. 2015.PMID:26187414Free PMC article.
- Distinct and Orchestrated Functions of RNA Sensors in Innate Immunity.Liu G, Gack MU.Liu G, et al.Immunity. 2020 Jul 14;53(1):26-42. doi: 10.1016/j.immuni.2020.03.017.Immunity. 2020.PMID:32668226Free PMC article.Review.
- 2-5A-Mediated decay (2-5AMD): from antiviral defense to control of host RNA.Prangley E, Korennykh A.Prangley E, et al.Crit Rev Biochem Mol Biol. 2022 Oct-Dec;57(5-6):477-491. doi: 10.1080/10409238.2023.2181308. Epub 2023 Mar 20.Crit Rev Biochem Mol Biol. 2022.PMID:36939319Free PMC article.Review.
- Interferon induction by RNA viruses and antagonism by viral pathogens.Nan Y, Nan G, Zhang YJ.Nan Y, et al.Viruses. 2014 Dec 12;6(12):4999-5027. doi: 10.3390/v6124999.Viruses. 2014.PMID:25514371Free PMC article.Review.
- Expression, purification and characterization of the interferon-inducible, antiviral and tumour-suppressor protein, human RNase L.Gupta A, Rath PC.Gupta A, et al.J Biosci. 2012 Mar;37(1):103-13. doi: 10.1007/s12038-011-9180-4.J Biosci. 2012.PMID:22357208
References
- Sato M, et al. Positive feedback regulation of type I IFN genes by the IFN-inducible transcription factor IRF-7. FEBS Lett. 1998;441:106–110. - PubMed
- Akira S, Uematsu S, Takeuchi O. Pathogen recognition and innate immunity. Cell. 2006;124:783–801. - PubMed
- Kawai T, Akira S. Innate immune recognition of viral infection. Nat Immunol. 2006;7:131–137. - PubMed
Publication types
MeSH terms
Substances
Related information
Grants and funding
LinkOut - more resources
Full Text Sources
Other Literature Sources
Miscellaneous