An integrated network of androgen receptor, polycomb, and TMPRSS2-ERG gene fusions in prostate cancer progression
- PMID:20478527
- PMCID: PMC2874722
- DOI: 10.1016/j.ccr.2010.03.018
An integrated network of androgen receptor, polycomb, and TMPRSS2-ERG gene fusions in prostate cancer progression
Abstract
Chromosomal rearrangements fusing the androgen-regulated gene TMPRSS2 to the oncogenic ETS transcription factor ERG occur in approximately 50% of prostate cancers, but how the fusion products regulate prostate cancer remains unclear. Using chromatin immunoprecipitation coupled with massively parallel sequencing, we found that ERG disrupts androgen receptor (AR) signaling by inhibiting AR expression, binding to and inhibiting AR activity at gene-specific loci, and inducing repressive epigenetic programs via direct activation of the H3K27 methyltransferase EZH2, a Polycomb group protein. These findings provide a working model in which TMPRSS2-ERG plays a critical role in cancer progression by disrupting lineage-specific differentiation of the prostate and potentiating the EZH2-mediated dedifferentiation program.
(c) 2010 Elsevier Inc. All rights reserved.
Figures
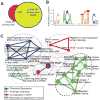
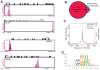
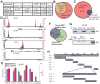
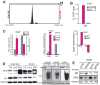
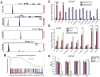
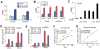
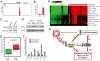
Comment in
- Coordinate transcriptional regulation by ERG and androgen receptor in fusion-positive prostate cancers.Chen Y, Sawyers CL.Chen Y, et al.Cancer Cell. 2010 May 18;17(5):415-6. doi: 10.1016/j.ccr.2010.04.022.Cancer Cell. 2010.PMID:20478521Free PMC article.
Similar articles
- TMPRSS2:ERG fusion by translocation or interstitial deletion is highly relevant in androgen-dependent prostate cancer, but is bypassed in late-stage androgen receptor-negative prostate cancer.Hermans KG, van Marion R, van Dekken H, Jenster G, van Weerden WM, Trapman J.Hermans KG, et al.Cancer Res. 2006 Nov 15;66(22):10658-63. doi: 10.1158/0008-5472.CAN-06-1871.Cancer Res. 2006.PMID:17108102
- Relation of ETS transcription factor family member ERG, androgen receptor and topoisomerase 2β expression to TMPRSS2-ERG fusion status in prostate cancer.Kolar Z, Burdova A, Jamaspishvili T, Bouchal J, Kucerova R, Bienova M, Kral M, Student V.Kolar Z, et al.Neoplasma. 2014;61(1):9-16.Neoplasma. 2014.PMID:24195515
- Binding of TMPRSS2-ERG to BAF Chromatin Remodeling Complexes Mediates Prostate Oncogenesis.Sandoval GJ, Pulice JL, Pakula H, Schenone M, Takeda DY, Pop M, Boulay G, Williamson KE, McBride MJ, Pan J, St Pierre R, Hartman E, Garraway LA, Carr SA, Rivera MN, Li Z, Ronco L, Hahn WC, Kadoch C.Sandoval GJ, et al.Mol Cell. 2018 Aug 16;71(4):554-566.e7. doi: 10.1016/j.molcel.2018.06.040. Epub 2018 Aug 2.Mol Cell. 2018.PMID:30078722Free PMC article.
- Emerging biological observations in prostate cancer.Shah S, Small E.Shah S, et al.Expert Rev Anticancer Ther. 2010 Jan;10(1):89-101. doi: 10.1586/era.09.161.Expert Rev Anticancer Ther. 2010.PMID:20014889Review.
- [Molecular aspects of prostate cancer: recent data from the literature].Camparo P, Vieillefond A.Camparo P, et al.Bull Cancer. 2007 Jul;94(7 Suppl):F77-88.Bull Cancer. 2007.PMID:17845997Review.French.
Cited by
- Modeling the Mechanism of GR/c-Jun/Erg Crosstalk in Apoptosis of Acute Lymphoblastic Leukemia.Chen DW, Krstic-Demonacos M, Schwartz JM.Chen DW, et al.Front Physiol. 2012 Nov 19;3:410. doi: 10.3389/fphys.2012.00410. eCollection 2012.Front Physiol. 2012.PMID:23181019Free PMC article.
- Molecular and pathological subtypes related to prostate cancer disparities and disease outcomes in African American and European American patients.Mori JO, White J, Elhussin I, Duduyemi BM, Karanam B, Yates C, Wang H.Mori JO, et al.Front Oncol. 2022 Aug 10;12:928357. doi: 10.3389/fonc.2022.928357. eCollection 2022.Front Oncol. 2022.PMID:36033462Free PMC article.
- Retinoic acid and androgen receptors combine to achieve tissue specific control of human prostatic transglutaminase expression: a novel regulatory network with broader significance.Rivera-Gonzalez GC, Droop AP, Rippon HJ, Tiemann K, Pellacani D, Georgopoulos LJ, Maitland NJ.Rivera-Gonzalez GC, et al.Nucleic Acids Res. 2012 Jun;40(11):4825-40. doi: 10.1093/nar/gks143. Epub 2012 Feb 22.Nucleic Acids Res. 2012.PMID:22362749Free PMC article.
- Oncogene-mediated alterations in chromatin conformation.Rickman DS, Soong TD, Moss B, Mosquera JM, Dlabal J, Terry S, MacDonald TY, Tripodi J, Bunting K, Najfeld V, Demichelis F, Melnick AM, Elemento O, Rubin MA.Rickman DS, et al.Proc Natl Acad Sci U S A. 2012 Jun 5;109(23):9083-8. doi: 10.1073/pnas.1112570109. Epub 2012 May 21.Proc Natl Acad Sci U S A. 2012.PMID:22615383Free PMC article.
- ERG induces epigenetic activation of Tudor domain-containing protein 1 (TDRD1) in ERG rearrangement-positive prostate cancer.Kacprzyk LA, Laible M, Andrasiuk T, Brase JC, Börno ST, Fälth M, Kuner R, Lehrach H, Schweiger MR, Sültmann H.Kacprzyk LA, et al.PLoS One. 2013;8(3):e59976. doi: 10.1371/journal.pone.0059976. Epub 2013 Mar 29.PLoS One. 2013.PMID:23555854Free PMC article.
References
- Anderson J. The role of antiandrogen monotherapy in the treatment of prostate cancer. BJU international. 2003;91:455–461. - PubMed
- Bailey TL, Elkan C. Fitting a mixture model by expectation maximization to discover motifs in biopolymers. Proceedings/International Conference on Intelligent Systems for Molecular Biology ; ISMB. 1994;2:28–36. - PubMed
- Barski A, Cuddapah S, Cui K, Roh TY, Schones DE, Wang Z, Wei G, Chepelev I, Zhao K. High-resolution profiling of histone methylations in the human genome. Cell. 2007;129:823–837. - PubMed
- Boyer LA, Plath K, Zeitlinger J, Brambrink T, Medeiros LA, Lee TI, Levine SS, Wernig M, Tajonar A, Ray MK, et al. Polycomb complexes repress developmental regulators in murine embryonic stem cells. Nature. 2006;441:349–353. - PubMed
Publication types
MeSH terms
Substances
Associated data
- Actions
Related information
Grants and funding
- K99CA129565-01A1/CA/NCI NIH HHS/United States
- U01 111275/PHS HHS/United States
- K99 CA129565-02/CA/NCI NIH HHS/United States
- K99 CA129565-02S1/CA/NCI NIH HHS/United States
- K99 CA129565/CA/NCI NIH HHS/United States
- P50 CA069568/CA/NCI NIH HHS/United States
- U01 CA111275/CA/NCI NIH HHS/United States
- P50CA090386/CA/NCI NIH HHS/United States
- R01 CA132874-01A1/CA/NCI NIH HHS/United States
- P50 CA069568-110020/CA/NCI NIH HHS/United States
- P50 CA069568-06A10016/CA/NCI NIH HHS/United States
- 1R01HG005119/HG/NHGRI NIH HHS/United States
- R01 CA132874/CA/NCI NIH HHS/United States
- U01 CA111275-01/CA/NCI NIH HHS/United States
- 1R01CA132874-01A1/CA/NCI NIH HHS/United States
- HHMI/Howard Hughes Medical Institute/United States
- P50CA69568/CA/NCI NIH HHS/United States
- P50 CA069568-06A19001/CA/NCI NIH HHS/United States
LinkOut - more resources
Full Text Sources
Other Literature Sources
Medical
Molecular Biology Databases
Research Materials