A systems approach to prion disease
- PMID:19308092
- PMCID: PMC2671916
- DOI: 10.1038/msb.2009.10
A systems approach to prion disease
Abstract
Prions cause transmissible neurodegenerative diseases and replicate by conformational conversion of normal benign forms of prion protein (PrP(C)) to disease-causing PrP(Sc) isoforms. A systems approach to disease postulates that disease arises from perturbation of biological networks in the relevant organ. We tracked global gene expression in the brains of eight distinct mouse strain-prion strain combinations throughout the progression of the disease to capture the effects of prion strain, host genetics, and PrP concentration on disease incubation time. Subtractive analyses exploiting various aspects of prion biology and infection identified a core of 333 differentially expressed genes (DEGs) that appeared central to prion disease. DEGs were mapped into functional pathways and networks reflecting defined neuropathological events and PrP(Sc) replication and accumulation, enabling the identification of novel modules and modules that may be involved in genetic effects on incubation time and in prion strain specificity. Our systems analysis provides a comprehensive basis for developing models for prion replication and disease, and suggests some possible therapeutic approaches.
Conflict of interest statement
The authors declare that they have no conflict of interest.
Figures
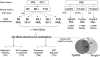
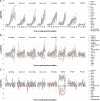
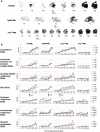
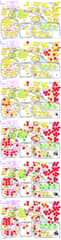
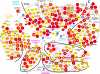
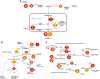
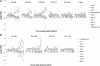
Comment in
- A landmark systems analysis of prion disease of the brain.Omenn GS.Omenn GS.Mol Syst Biol. 2009;5:254. doi: 10.1038/msb.2009.12. Epub 2009 Mar 24.Mol Syst Biol. 2009.PMID:19308093Free PMC article.No abstract available.
Similar articles
- Complement 3+-astrocytes are highly abundant in prion diseases, but their abolishment led to an accelerated disease course and early dysregulation of microglia.Hartmann K, Sepulveda-Falla D, Rose IVL, Madore C, Muth C, Matschke J, Butovsky O, Liddelow S, Glatzel M, Krasemann S.Hartmann K, et al.Acta Neuropathol Commun. 2019 May 22;7(1):83. doi: 10.1186/s40478-019-0735-1.Acta Neuropathol Commun. 2019.PMID:31118110Free PMC article.
- Flow Cytometric Detection of PrPSc in Neurons and Glial Cells from Prion-Infected Mouse Brains.Yamasaki T, Suzuki A, Hasebe R, Horiuchi M.Yamasaki T, et al.J Virol. 2017 Dec 14;92(1):e01457-17. doi: 10.1128/JVI.01457-17. Print 2018 Jan 1.J Virol. 2017.PMID:29046463Free PMC article.
- An astrocyte cell line that differentially propagates murine prions.Tahir W, Abdulrahman B, Abdelaziz DH, Thapa S, Walia R, Schätzl HM.Tahir W, et al.J Biol Chem. 2020 Aug 14;295(33):11572-11583. doi: 10.1074/jbc.RA120.012596. Epub 2020 Jun 19.J Biol Chem. 2020.PMID:32561641Free PMC article.
- Prion encephalopathies of animals and humans.Prusiner SB.Prusiner SB.Dev Biol Stand. 1993;80:31-44.Dev Biol Stand. 1993.PMID:8270114Review.
- Genetics of prion diseases and prion diversity in mice.Carlson GA, DeArmond SJ, Torchia M, Westaway D, Prusiner SB.Carlson GA, et al.Philos Trans R Soc Lond B Biol Sci. 1994 Mar 29;343(1306):363-9. doi: 10.1098/rstb.1994.0030.Philos Trans R Soc Lond B Biol Sci. 1994.PMID:7913753Review.
Cited by
- Systems approaches to biology and disease enable translational systems medicine.Hood L, Tian Q.Hood L, et al.Genomics Proteomics Bioinformatics. 2012 Aug;10(4):181-5. doi: 10.1016/j.gpb.2012.08.004. Epub 2012 Aug 23.Genomics Proteomics Bioinformatics. 2012.PMID:23084773Free PMC article.Review.
- A Bacterial Component to Alzheimer's-Type Dementia Seen via a Systems Biology Approach that Links Iron Dysregulation and Inflammagen Shedding to Disease.Pretorius E, Bester J, Kell DB.Pretorius E, et al.J Alzheimers Dis. 2016 Jun 18;53(4):1237-56. doi: 10.3233/JAD-160318.J Alzheimers Dis. 2016.PMID:27340854Free PMC article.Review.
- Three-dimensional reconstruction of protein networks provides insight into human genetic disease.Wang X, Wei X, Thijssen B, Das J, Lipkin SM, Yu H.Wang X, et al.Nat Biotechnol. 2012 Jan 15;30(2):159-64. doi: 10.1038/nbt.2106.Nat Biotechnol. 2012.PMID:22252508Free PMC article.
- Prion disease and the innate immune system.Bradford BM, Mabbott NA.Bradford BM, et al.Viruses. 2012 Dec;4(12):3389-419. doi: 10.3390/v4123389.Viruses. 2012.PMID:23342365Free PMC article.Review.
- Quaternary Structure Changes for PrPSc Predate PrPC Downregulation and Neuronal Death During Progression of Experimental Scrapie Disease.Eskandari-Sedighi G, Cortez LM, Yang J, Daude N, Shmeit K, Sim V, Westaway D.Eskandari-Sedighi G, et al.Mol Neurobiol. 2021 Jan;58(1):375-390. doi: 10.1007/s12035-020-02112-z. Epub 2020 Sep 21.Mol Neurobiol. 2021.PMID:32959170Free PMC article.
References
- Ben-Zaken O, Tzaban S, Tal Y, Horonchik L, Esko JD, Vlodavsky I, Taraboulos A (2003) Cellular heparan sulfate participates in the metabolism of prions. J Biol Chem 278: 40041–40049 - PubMed
- Berriz GF, King OD, Bryant B, Sander C, Roth FP (2003) Characterizing gene sets with FuncAssociate. Bioinformatics 19: 2502–2504 - PubMed
Publication types
MeSH terms
Substances
Related information
Grants and funding
LinkOut - more resources
Full Text Sources
Other Literature Sources
Molecular Biology Databases
Research Materials