Miscibility and in vitro osteocompatibility of biodegradable blends of poly[(ethyl alanato) (p-phenyl phenoxy) phosphazene] and poly(lactic acid-glycolic acid)
- PMID:17942150
- PMCID: PMC2129129
- DOI: 10.1016/j.biomaterials.2007.09.029
Miscibility and in vitro osteocompatibility of biodegradable blends of poly[(ethyl alanato) (p-phenyl phenoxy) phosphazene] and poly(lactic acid-glycolic acid)
Abstract
Previously we demonstrated the ability of ethyl glycinato substituted polyphosphazenes to neutralize the acidic degradation products and control the degradation rate of poly(lactic acid-glycolic acid) (PLAGA) by blending. In this study, blends of high strength poly[(50% ethyl alanato) (50% p-phenyl phenoxy) phosphazene] (PNEA(50)PhPh(50)) and 85:15 PLAGA were prepared using a mutual solvent approach. Three different solvents, methylene chloride (MC), chloroform (CF) and tetrahydrofuran (THF) were studied to investigate solvent effects on blend miscibility. Three different blends were then fabricated at various weight ratios namely 25:75 (BLEND25), 50:50 (BLEND50), and 75:25 (BLEND75) using THF as the mutual solvent. The miscibility of the blends was evaluated by scanning electron microscopy (SEM), differential scanning calorimetry (DSC), and Fourier transform infrared spectroscopy (FTIR). Among these, BLEND25 was miscible while BLEND50 and BLEND75 were partially miscible. Furthermore, BLEND25 formed apatite layers on its surface as evidenced in a biomimetic study performed. These novel blends showed cell adhesion and proliferation comparable to PLAGA. However, the PNEA(50)PhPh(50) component in the blends was able to increase the phenotypic expression and mineralized matrix synthesis of the primary rat osteoblasts (PRO) in vitro. Blends of high strength PNEA(50)PhPh(50) and 85:15 PLAGA are promising biomaterials for a variety of musculoskeletal applications.
Figures
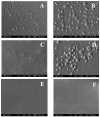
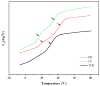
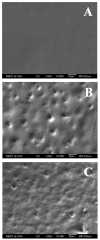
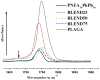
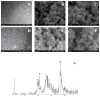
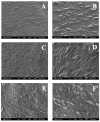
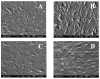
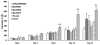
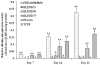
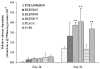
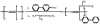
Similar articles
- Dipeptide-based polyphosphazene and polyester blends for bone tissue engineering.Deng M, Nair LS, Nukavarapu SP, Jiang T, Kanner WA, Li X, Kumbar SG, Weikel AL, Krogman NR, Allcock HR, Laurencin CT.Deng M, et al.Biomaterials. 2010 Jun;31(18):4898-908. doi: 10.1016/j.biomaterials.2010.02.058. Epub 2010 Mar 23.Biomaterials. 2010.PMID:20334909Free PMC article.
- Novel polyphosphazene/poly(lactide-co-glycolide) blends: miscibility and degradation studies.Ibim SE, Ambrosio AM, Kwon MS, El-Amin SF, Allcock HR, Laurencin CT.Ibim SE, et al.Biomaterials. 1997 Dec;18(23):1565-9. doi: 10.1016/s0142-9612(97)80009-9.Biomaterials. 1997.PMID:9430340
- Mechanical properties and osteocompatibility of novel biodegradable alanine based polyphosphazenes: Side group effects.Sethuraman S, Nair LS, El-Amin S, Nguyen MT, Singh A, Krogman N, Greish YE, Allcock HR, Brown PW, Laurencin CT.Sethuraman S, et al.Acta Biomater. 2010 Jun;6(6):1931-7. doi: 10.1016/j.actbio.2009.12.012. Epub 2009 Dec 24.Acta Biomater. 2010.PMID:20004751Free PMC article.
- Poly(lactic acid) blends in biomedical applications.Saini P, Arora M, Kumar MNVR.Saini P, et al.Adv Drug Deliv Rev. 2016 Dec 15;107:47-59. doi: 10.1016/j.addr.2016.06.014. Epub 2016 Jun 29.Adv Drug Deliv Rev. 2016.PMID:27374458Review.
- Recent advances in synthetic bioelastomers.Shi R, Chen D, Liu Q, Wu Y, Xu X, Zhang L, Tian W.Shi R, et al.Int J Mol Sci. 2009 Nov 20;10(10):4223-4256. doi: 10.3390/ijms10104223.Int J Mol Sci. 2009.PMID:20057942Free PMC article.Review.
Cited by
- POLYMERIC BIOMATERIALS FOR SCAFFOLD-BASED BONE REGENERATIVE ENGINEERING.Ogueri KS, Jafari T, Escobar Ivirico JL, Laurencin CT.Ogueri KS, et al.Regen Eng Transl Med. 2019 Jun;5(2):128-154. doi: 10.1007/s40883-018-0072-0. Epub 2018 Jul 20.Regen Eng Transl Med. 2019.PMID:31423461Free PMC article.
- Dipeptide-based polyphosphazene and polyester blends for bone tissue engineering.Deng M, Nair LS, Nukavarapu SP, Jiang T, Kanner WA, Li X, Kumbar SG, Weikel AL, Krogman NR, Allcock HR, Laurencin CT.Deng M, et al.Biomaterials. 2010 Jun;31(18):4898-908. doi: 10.1016/j.biomaterials.2010.02.058. Epub 2010 Mar 23.Biomaterials. 2010.PMID:20334909Free PMC article.
- Biomedical Applications of Biodegradable Polymers.Ulery BD, Nair LS, Laurencin CT.Ulery BD, et al.J Polym Sci B Polym Phys. 2011 Jun 15;49(12):832-864. doi: 10.1002/polb.22259.J Polym Sci B Polym Phys. 2011.PMID:21769165Free PMC article.
- Self-neutralizing PLGA/magnesium composites as novel biomaterials for tissue engineering.Xu TO, Kim HS, Stahl T, Nukavarapu SP.Xu TO, et al.Biomed Mater. 2018 Mar 16;13(3):035013. doi: 10.1088/1748-605X/aaaa29.Biomed Mater. 2018.PMID:29362293Free PMC article.
- Inductive Materials for Regenerative Engineering.Hosseini FS, Nair LS, Laurencin CT.Hosseini FS, et al.J Dent Res. 2021 Sep;100(10):1011-1019. doi: 10.1177/00220345211010436. Epub 2021 Apr 27.J Dent Res. 2021.PMID:33906507Free PMC article.Review.
References
- Langer R, Tirrell DA. Designing materials for biology and medicine. Nature. 2004;428:487–492. - PubMed
- Hubbell JA. Biomaterials in Tissue Engineering. Nat Biotech. 1995;13:565–576. - PubMed
- Burg KJL, Porter S, Kellam JF. Biomaterial developments for bone tissue engineering. Biomaterials. 2000;21:2347–2359. - PubMed
- Ratner BD, Bryant SJ. BIOMATERIALS: Where We Have Been and Where We are Going. Annu Rev Biomed Eng. 2004;6:41–75. - PubMed
- Vacanti JP, Vacanti CA. The history and scope of tissue engineering. In: Lanza RP, Langer R, Vacanti JP, editors. Principles of Tissue Engineering. New York: Academic Press; 2000. pp. 3–8.
Publication types
MeSH terms
Substances
Related information
Grants and funding
LinkOut - more resources
Full Text Sources
Other Literature Sources