G protein-coupled receptor rhodopsin
- PMID:16756510
- PMCID: PMC1560097
- DOI: 10.1146/annurev.biochem.75.103004.142743
G protein-coupled receptor rhodopsin
Abstract
The rhodopsin crystal structure provides a structural basis for understanding the function of this and other G protein-coupled receptors (GPCRs). The major structural motifs observed for rhodopsin are expected to carry over to other GPCRs, and the mechanism of transformation of the receptor from inactive to active forms is thus likely conserved. Moreover, the high expression level of rhodopsin in the retina, its specific localization in the internal disks of the photoreceptor structures [termed rod outer segments (ROS)], and the lack of other highly abundant membrane proteins allow rhodopsin to be examined in the native disk membranes by a number of methods. The results of these investigations provide evidence of the propensity of rhodopsin and, most likely, other GPCRs to dimerize, a property that may be pertinent to their function.
Figures
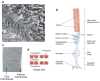
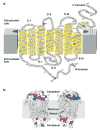
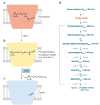
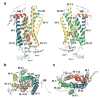
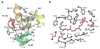
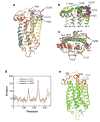
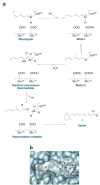
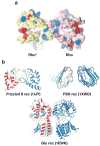
Similar articles
- The G protein-coupled receptor rhodopsin in the native membrane.Fotiadis D, Liang Y, Filipek S, Saperstein DA, Engel A, Palczewski K.Fotiadis D, et al.FEBS Lett. 2004 Apr 30;564(3):281-288. doi: 10.1016/S0014-5793(04)00194-2.FEBS Lett. 2004.PMID:15111110Free PMC article.
- Structure of the rhodopsin dimer: a working model for G-protein-coupled receptors.Fotiadis D, Jastrzebska B, Philippsen A, Müller DJ, Palczewski K, Engel A.Fotiadis D, et al.Curr Opin Struct Biol. 2006 Apr;16(2):252-9. doi: 10.1016/j.sbi.2006.03.013. Epub 2006 Mar 29.Curr Opin Struct Biol. 2006.PMID:16567090Review.
- The supramolecular structure of the GPCR rhodopsin in solution and native disc membranes.Suda K, Filipek S, Palczewski K, Engel A, Fotiadis D.Suda K, et al.Mol Membr Biol. 2004 Nov-Dec;21(6):435-46. doi: 10.1080/09687860400020291.Mol Membr Biol. 2004.PMID:15764373Free PMC article.
- The role of cholesterol in rod outer segment membranes.Albert AD, Boesze-Battaglia K.Albert AD, et al.Prog Lipid Res. 2005 Mar-May;44(2-3):99-124. doi: 10.1016/j.plipres.2005.02.001. Epub 2005 Mar 9.Prog Lipid Res. 2005.PMID:15924998Free PMC article.Review.
- Agonist-induced conformational changes in bovine rhodopsin: insight into activation of G-protein-coupled receptors.Bhattacharya S, Hall SE, Vaidehi N.Bhattacharya S, et al.J Mol Biol. 2008 Oct 3;382(2):539-55. doi: 10.1016/j.jmb.2008.06.084. Epub 2008 Jul 7.J Mol Biol. 2008.PMID:18638482
Cited by
- Structural and functional protein network analyses predict novel signaling functions for rhodopsin.Kiel C, Vogt A, Campagna A, Chatr-aryamontri A, Swiatek-de Lange M, Beer M, Bolz S, Mack AF, Kinkl N, Cesareni G, Serrano L, Ueffing M.Kiel C, et al.Mol Syst Biol. 2011 Nov 22;7:551. doi: 10.1038/msb.2011.83.Mol Syst Biol. 2011.PMID:22108793Free PMC article.
- Tuning the electronic absorption of protein-embedded all-trans-retinal.Wang W, Nossoni Z, Berbasova T, Watson CT, Yapici I, Lee KS, Vasileiou C, Geiger JH, Borhan B.Wang W, et al.Science. 2012 Dec 7;338(6112):1340-3. doi: 10.1126/science.1226135.Science. 2012.PMID:23224553Free PMC article.
- Structural view of G protein-coupled receptor signaling in the retinal rod outer segment.Gulati S, Palczewski K.Gulati S, et al.Trends Biochem Sci. 2023 Feb;48(2):172-186. doi: 10.1016/j.tibs.2022.08.010. Epub 2022 Sep 23.Trends Biochem Sci. 2023.PMID:36163145Free PMC article.Review.
- Human cone elongation responses can be explained by photoactivated cone opsin and membrane swelling and osmotic response to phosphate produced by RGS9-catalyzed GTPase.Pandiyan VP, Nguyen PT, Pugh EN Jr, Sabesan R.Pandiyan VP, et al.Proc Natl Acad Sci U S A. 2022 Sep 27;119(39):e2202485119. doi: 10.1073/pnas.2202485119. Epub 2022 Sep 19.Proc Natl Acad Sci U S A. 2022.PMID:36122241Free PMC article.
- Retina, retinol, retinal and the natural history of vitamin A as a light sensor.Zhong M, Kawaguchi R, Kassai M, Sun H.Zhong M, et al.Nutrients. 2012 Dec 19;4(12):2069-96. doi: 10.3390/nu4122069.Nutrients. 2012.PMID:23363998Free PMC article.Review.
References
Publication types
MeSH terms
Substances
Related information
Grants and funding
LinkOut - more resources
Full Text Sources
Other Literature Sources
Medical