Lessons from lactose permease
- PMID:16689628
- PMCID: PMC2802108
- DOI: 10.1146/annurev.biophys.35.040405.102005
Lessons from lactose permease
Abstract
An X-ray structure of the lactose permease of Escherichia coli (LacY) in an inward-facing conformation has been solved. LacY contains N- and C-terminal domains, each with six transmembrane helices, positioned pseudosymmetrically. Ligand is bound at the apex of a hydrophilic cavity in the approximate middle of the molecule. Residues involved in substrate binding and H+ translocation are aligned parallel to the membrane at the same level and may be exposed to a water-filled cavity in both the inward- and outward-facing conformations, thereby allowing both sugar and H+ release directly into either cavity. These structural features may explain why LacY catalyzes galactoside/H+ symport in both directions utilizing the same residues. A working model for the mechanism is presented that involves alternating access of both the sugar- and H+-binding sites to either side of the membrane.
Figures
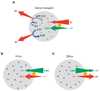
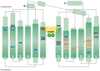
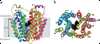
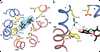
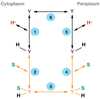
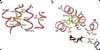
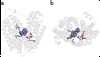
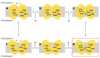
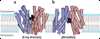
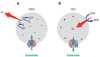
Similar articles
- The alternating access transport mechanism in LacY.Kaback HR, Smirnova I, Kasho V, Nie Y, Zhou Y.Kaback HR, et al.J Membr Biol. 2011 Jan;239(1-2):85-93. doi: 10.1007/s00232-010-9327-5. Epub 2010 Dec 16.J Membr Biol. 2011.PMID:21161516Free PMC article.Review.
- Substrate-induced changes in the structural properties of LacY.Serdiuk T, Madej MG, Sugihara J, Kawamura S, Mari SA, Kaback HR, Müller DJ.Serdiuk T, et al.Proc Natl Acad Sci U S A. 2014 Apr 22;111(16):E1571-80. doi: 10.1073/pnas.1404446111. Epub 2014 Apr 7.Proc Natl Acad Sci U S A. 2014.PMID:24711390Free PMC article.
- Lactose permease and the alternating access mechanism.Smirnova I, Kasho V, Kaback HR.Smirnova I, et al.Biochemistry. 2011 Nov 15;50(45):9684-93. doi: 10.1021/bi2014294. Epub 2011 Oct 19.Biochemistry. 2011.PMID:21995338Free PMC article.
- Opening the periplasmic cavity in lactose permease is the limiting step for sugar binding.Smirnova I, Kasho V, Sugihara J, Kaback HR.Smirnova I, et al.Proc Natl Acad Sci U S A. 2011 Sep 13;108(37):15147-51. doi: 10.1073/pnas.1112157108. Epub 2011 Sep 6.Proc Natl Acad Sci U S A. 2011.PMID:21896727Free PMC article.
- It takes two to tango: The dance of the permease.Kaback HR, Guan L.Kaback HR, et al.J Gen Physiol. 2019 Jul 1;151(7):878-886. doi: 10.1085/jgp.201912377. Epub 2019 May 30.J Gen Physiol. 2019.PMID:31147449Free PMC article.Review.
Cited by
- Crystal structure of a bacterial homologue of glucose transporters GLUT1-4.Sun L, Zeng X, Yan C, Sun X, Gong X, Rao Y, Yan N.Sun L, et al.Nature. 2012 Oct 18;490(7420):361-6. doi: 10.1038/nature11524.Nature. 2012.PMID:23075985
- Crystal structure of a LacY-nanobody complex in a periplasmic-open conformation.Jiang X, Smirnova I, Kasho V, Wu J, Hirata K, Ke M, Pardon E, Steyaert J, Yan N, Kaback HR.Jiang X, et al.Proc Natl Acad Sci U S A. 2016 Nov 1;113(44):12420-12425. doi: 10.1073/pnas.1615414113. Epub 2016 Oct 19.Proc Natl Acad Sci U S A. 2016.PMID:27791182Free PMC article.
- Structural determination of wild-type lactose permease.Guan L, Mirza O, Verner G, Iwata S, Kaback HR.Guan L, et al.Proc Natl Acad Sci U S A. 2007 Sep 25;104(39):15294-8. doi: 10.1073/pnas.0707688104. Epub 2007 Sep 19.Proc Natl Acad Sci U S A. 2007.PMID:17881559Free PMC article.
- Direct sugar binding to LacY measured by resonance energy transfer.Smirnova IN, Kasho VN, Kaback HR.Smirnova IN, et al.Biochemistry. 2006 Dec 26;45(51):15279-87. doi: 10.1021/bi061632m. Epub 2006 Nov 29.Biochemistry. 2006.PMID:17176050Free PMC article.
- Functional architecture of MFS D-glucose transporters.Madej MG, Sun L, Yan N, Kaback HR.Madej MG, et al.Proc Natl Acad Sci U S A. 2014 Feb 18;111(7):E719-27. doi: 10.1073/pnas.1400336111. Epub 2014 Feb 3.Proc Natl Acad Sci U S A. 2014.PMID:24550316Free PMC article.
References
- Abramson J, Kaback HR, Iwata S. Structural comparison of lactose permease and the glycerol-3-phosphate antiporter: members of the major facilitator superfamily. Curr. Opin. Struct. Biol. 2004;14:413–419. - PubMed
- Abramson J, Smirnova I, Kasho V, Verner G, Kaback HR, Iwata S. Structure and mechanism of the lactose permease of Escherichia coli. Science. 2003;301:610–615. - PubMed
- Bieseler B, Prinz H, Beyreuther K. Topological studies of lactose permease of Escherichia coli by protein sequence analysis. Ann. N. Y. Acad. Sci. 1985;456:309–325. - PubMed
Publication types
MeSH terms
Substances
Related information
Grants and funding
- R01 DK051131-12/DK/NIDDK NIH HHS/United States
- R01 DK051131-06/DK/NIDDK NIH HHS/United States
- U54 GM074929-030005/GM/NIGMS NIH HHS/United States
- R01 DK051131-07/DK/NIDDK NIH HHS/United States
- R01 DK069463-01/DK/NIDDK NIH HHS/United States
- R01 DK051131-04/DK/NIDDK NIH HHS/United States
- R56 DK069463-05A1/DK/NIDDK NIH HHS/United States
- P50 GM073210/GM/NIGMS NIH HHS/United States
- U54 GM074929-040005/GM/NIGMS NIH HHS/United States
- R01 DK051131-05/DK/NIDDK NIH HHS/United States
- R01 DK051131-09/DK/NIDDK NIH HHS/United States
- R01 DK069463-03/DK/NIDDK NIH HHS/United States
- R56 DK051131/DK/NIDDK NIH HHS/United States
- U54 GM074929-050005/GM/NIGMS NIH HHS/United States
- R01 DK051131-13/DK/NIDDK NIH HHS/United States
- R01 DK051131-11/DK/NIDDK NIH HHS/United States
- R01 DK069463-02/DK/NIDDK NIH HHS/United States
- R01 DK051131-14/DK/NIDDK NIH HHS/United States
- R13 DK058527-01/DK/NIDDK NIH HHS/United States
- R01 DK051131/DK/NIDDK NIH HHS/United States
- U54 GM074929-010005/GM/NIGMS NIH HHS/United States
- R01 DK051131-10/DK/NIDDK NIH HHS/United States
- R01 DK051131-08/DK/NIDDK NIH HHS/United States
- U54 GM074929-020005/GM/NIGMS NIH HHS/United States
- U54 GM074929/GM/NIGMS NIH HHS/United States
- R01 DK069463-04/DK/NIDDK NIH HHS/United States
- R56 DK069463/DK/NIDDK NIH HHS/United States
- R01 DK069463/DK/NIDDK NIH HHS/United States
LinkOut - more resources
Full Text Sources
Other Literature Sources
Molecular Biology Databases
Miscellaneous