Implication of proprotein convertases in the processing and spread of severe acute respiratory syndrome coronavirus
- PMID:15596135
- PMCID: PMC7092861
- DOI: 10.1016/j.bbrc.2004.11.063
Implication of proprotein convertases in the processing and spread of severe acute respiratory syndrome coronavirus
Abstract
Severe acute respiratory syndrome coronavirus (SARS-CoV) is the etiological agent of SARS. Analysis of SARS-CoV spike glycoprotein (S) using recombinant plasmid and virus infections demonstrated that the S-precursor (proS) exists as a approximately 190 kDa endoplasmic reticulum form and a approximately 210 kDa Golgi-modified form. ProS is subsequently processed into two C-terminal proteins of approximately 110 and approximately 80 kDa. The membrane-bound proprotein convertases (PCs) furin, PC7 or PC5B enhanced the production of the approximately 80 kDa protein. In agreement, proS processing, cytopathic effects, and viral titers were enhanced in recombinant Vero E6 cells overexpressing furin, PC7 or PC5B. The convertase inhibitor dec-RVKR-cmk significantly reduced proS cleavage and viral titers of SARS-CoV infected cells. In addition, inhibition of processing by dec-RVKR-cmk completely abrogated the virus-induced cellular cytopathicity. A fluorogenically quenched synthetic peptide encompassing Arg(761) of the spike glycoprotein was efficiently cleaved by furin and the cleavage was inhibited by EDTA and dec-RVKR-cmk. Taken together, our data indicate that furin or PC-mediated processing plays a critical role in SARS-CoV spread and cytopathicity, and inhibitors of the PCs represent potential therapeutic anti-SARS-CoV agents.
Figures
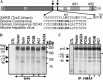
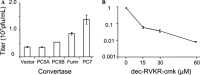
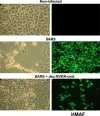
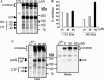
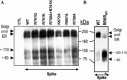
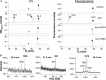
Similar articles
- Middle East Respiratory Syndrome Coronavirus Spike Protein Is Not Activated Directly by Cellular Furin during Viral Entry into Target Cells.Matsuyama S, Shirato K, Kawase M, Terada Y, Kawachi K, Fukushi S, Kamitani W.Matsuyama S, et al.J Virol. 2018 Sep 12;92(19):e00683-18. doi: 10.1128/JVI.00683-18. Print 2018 Oct 1.J Virol. 2018.PMID:30021905Free PMC article.
- Neuroinflammation-Induced Interactions between Protease-Activated Receptor 1 and Proprotein Convertases in HIV-Associated Neurocognitive Disorder.Kim W, Zekas E, Lodge R, Susan-Resiga D, Marcinkiewicz E, Essalmani R, Mihara K, Ramachandran R, Asahchop E, Gelman B, Cohen ÉA, Power C, Hollenberg MD, Seidah NG.Kim W, et al.Mol Cell Biol. 2015 Nov;35(21):3684-700. doi: 10.1128/MCB.00764-15. Epub 2015 Aug 17.Mol Cell Biol. 2015.PMID:26283733Free PMC article.
- The regulated cell surface zymogen activation of the proprotein convertase PC5A directs the processing of its secretory substrates.Mayer G, Hamelin J, Asselin MC, Pasquato A, Marcinkiewicz E, Tang M, Tabibzadeh S, Seidah NG.Mayer G, et al.J Biol Chem. 2008 Jan 25;283(4):2373-84. doi: 10.1074/jbc.M708763200. Epub 2007 Nov 26.J Biol Chem. 2008.PMID:18039650
- How Do Enveloped Viruses Exploit the Secretory Proprotein Convertases to Regulate Infectivity and Spread?Seidah NG, Pasquato A, Andréo U.Seidah NG, et al.Viruses. 2021 Jun 25;13(7):1229. doi: 10.3390/v13071229.Viruses. 2021.PMID:34202098Free PMC article.Review.
- Knock-out mouse models of proprotein convertases: unique functions or redundancy?Creemers JW, Khatib AM.Creemers JW, et al.Front Biosci. 2008 May 1;13:4960-71. doi: 10.2741/3055.Front Biosci. 2008.PMID:18508561Review.
Cited by
- Cutting back on pro-protein convertases: the latest approaches to pharmacological inhibition.Fugère M, Day R.Fugère M, et al.Trends Pharmacol Sci. 2005 Jun;26(6):294-301. doi: 10.1016/j.tips.2005.04.006.Trends Pharmacol Sci. 2005.PMID:15925704Free PMC article.Review.
- Cell-surface processing of extracellular human immunodeficiency virus type 1 Vpr by proprotein convertases.Xiao Y, Chen G, Richard J, Rougeau N, Li H, Seidah NG, Cohen EA.Xiao Y, et al.Virology. 2008 Mar 15;372(2):384-97. doi: 10.1016/j.virol.2007.10.036. Epub 2007 Dec 3.Virology. 2008.PMID:18061232Free PMC article.
- Union is strength: antiviral and anti-inflammatory drugs for COVID-19.Naveja JJ, Madariaga-Mazón A, Flores-Murrieta F, Granados-Montiel J, Maradiaga-Ceceña M, Alaniz VD, Maldonado-Rodriguez M, García-Morales J, Senosiain-Peláez JP, Martinez-Mayorga K.Naveja JJ, et al.Drug Discov Today. 2021 Jan;26(1):229-239. doi: 10.1016/j.drudis.2020.10.018. Epub 2020 Oct 27.Drug Discov Today. 2021.PMID:33127568Free PMC article.Review.
- Adenovirus-mediated expression of the C-terminal domain of SARS-CoV spike protein is sufficient to induce apoptosis in Vero E6 cells.Chow KY, Yeung YS, Hon CC, Zeng F, Law KM, Leung FC.Chow KY, et al.FEBS Lett. 2005 Dec 19;579(30):6699-704. doi: 10.1016/j.febslet.2005.10.065. Epub 2005 Nov 21.FEBS Lett. 2005.PMID:16310778Free PMC article.
- Evidence that TMPRSS2 activates the severe acute respiratory syndrome coronavirus spike protein for membrane fusion and reduces viral control by the humoral immune response.Glowacka I, Bertram S, Müller MA, Allen P, Soilleux E, Pfefferle S, Steffen I, Tsegaye TS, He Y, Gnirss K, Niemeyer D, Schneider H, Drosten C, Pöhlmann S.Glowacka I, et al.J Virol. 2011 May;85(9):4122-34. doi: 10.1128/JVI.02232-10. Epub 2011 Feb 16.J Virol. 2011.PMID:21325420Free PMC article.
References
- Ksiazek T.G., Erdman D., Goldsmith C.S., Zaki S.R., Peret T., Emery S., Tong S., Urbani C., Comer J.A., Lim W., Rollin P.E, Dowell S.F., Ling A.E., Humphrey C.D., Shieh W.J., Guarner J., Paddock C.D., Rota P., Fields B., DeRisi J., Yang J.Y., Cox N., Hughes J.M., LeDuc J.W., Bellini W.J., Anderson L.J. N. Engl. J. Med. 2003;348:1953–1966. - PubMed
- Marra M.A., Jones S.J., Astell C.R., Holt R.A., Brooks-Wilson A., Butterfield Y.S., Khattra J., Asano J.K., Barber S.A., Chan S.Y., Cloutier A., Coughlin S.M., Freeman D., Girn N., Griffith O.L., Leach S.R., Mayo M., McDonald H., Montgomery S.B., Pandoh P.K., Petrescu A.S., Robertson A.G., Schein J.E., Siddiqui A., Smailus D.E., Stott J.M., Yang G.S., Plummer F., Andonov A., Artsob H., Bastien N., Bernard K., Booth T.F., Bowness D., Czub M., Drebot M., Fernando L., Flick R., Garbutt M., Gray M., Grolla A., Jones S., Feldmann H., Meyers A., Kabani A., Li Y., Normand S., Stroher U., Tipples G.A., Tyler S., Vogrig R., Ward D., Watson B., Brunham R.C., Krajden M., Petric M., Skowronski D.M., Upton C., Roper R.L. Science. 2003;300:1399–1404. - PubMed
- Rota P.A., Oberste M.S., Monroe S.S., Nix W.A., Campagnoli R., Icenogle J.P., Penaranda S., Bankamp B., Maher K., Chen M.H., Tong S., Tamin A., Lowe L., Frace M., DeRisi J.L., Chen Q., Wang D., Erdman D.D., Peret T.C., Burns C., Ksiazek T.G., Rollin P.E., Sanchez A., Liffick S., Holloway B., Limor J., McCaustland K., Olsen-Rasmussen M., Fouchier R., Gunther S., Osterhaus A.D., Drosten C., Pallansch M.A., Anderson L.J., Bellini W.J. Science. 2003;300:1394–1399. - PubMed
- Ng M.L., Tan S.H., See E.E., Ooi E.E., Ling A.E. J. Gen. Virol. 2003;84:3291–3303. - PubMed
Publication types
MeSH terms
Substances
Related information
LinkOut - more resources
Full Text Sources
Other Literature Sources
Research Materials
Miscellaneous