COMPOSITION AND METHODS FOR TREATMENT OF SPINAL CORD INJURY AND TRAUMATIC BRAIN INJURY
CROSS-REFERENCE TO RELATED APPLICATIONS
[0001] This application claims priority to United States provisional patent application number 63/461,985, which was filed on April 26, 2023.
SEQUENCE LISTING
[0002] This application incorporates by reference the material in the Extensible Markup Language (XML) file titled IUIC-00127ST26sequencelisting.xml, which is 2,080 bytes in size.
STATEMENT REGARDING FEDERALLY SPONSORED
RESEARCH OR DEVELOPMENT
[0003] This invention was made with government support under NS100531 and NS103481 awarded by the National Institutes of Health. The Government has certain rights in the invention.
BACKGROUND
[0004] The present disclosure relates generally to systems, materials, and methods for treatment of traumatic injury in a mammalian subject in need thereof.
[0005] Traumatic neurologic injuries such as spinal cord injury (SCI) and traumatic brain injury (TBI) represent a significant public health issue, affecting millions of people worldwide, with hundreds of thousands of new cases reported annually. Neurologic trauma is a common cause of permanent disability in children and adults, with a significant socioeconomic impact on affected individuals and the healthcare system.
[0006] Neurotrauma comprises primary and secondy injuries. The primary injury refers to the initial mechanical impact that causes focal and diffuse damage of the central nervous system (CNS), including, e.g., necrosis, hematomas, hemorrhages, and damage to axons, oligodendrocytes, and vasculature. Other than injury prevention, little can be done to intervene with the primary injury itself. Secondary injury is an indirect consequence of destructive immunological, inflammatory, neurotoxic, and biochemical cascades that are initially triggered by the primary injury. Excitotoxicity, mitochondrial dysfunction, oxidative stress, lipid peroxidation, neuroinflammation, axon degeneration, and apoptosis all seem to occur after a neurotraumatic injury, and contribute to secondary injury processes. See Ray S.K. et al., Molecular mechanisms in the pathogenesis of traumatic brain injury. Histol. Histopathol. 2002;17: 1137-52.
[0007] Neurotrauma secondary injury remains an important determinant of clinical outcome, as the damage associated with secondary injury can often be in excess of that caused by the primary injury. However, the mechanisms of the immune and inflammatory responses involved in secondary injury remain largely unknown. It is critical to unravel these mechanisms for developing effective treatments of devastating neurotraumatic secondary injury to fill a significant unmet medical need.
[0008] Accordingly, the present disclosure provides new and surprising systems, materials, and methods for treating trauma in a subject in need thereof.
SUMMARY
[0009] The present disclosure relates to systems, materials, and methods for treatment of traumatic injury in a mammalian subject in need thereof. The present disclosure further relates to improvements in therapeutic discovery processes, particularly for developing therapeutics that target CD Id.
[0010] In an aspect, the present disclosure provides a therapeutic composition for treatment of physical trauma, the therapeutic comprising an inhibitor of CDld. In some embodiments, the composition may comprise a small-molecule pharmaceutical. In some embodiments, the composition may comprise an antibody or antibody fragment. In such embodiments, the antibody or antibody fragment may comprise any of a F(ab')2, F(ab')2 fragment, Fab', F(ab)2, Fab, Fab fragment, Fv, sFv, single-chain variable fragment (scFv), single-domain antibody (sdAb), nanobody, diabody, triabody, minibody, or combination thereof. The antibody or antibody fragment may specifically bind to a CDld protein or peptide having at least 70%, at least 80%, at least 90%, or 100% sequence identity to SEQ ID NO: 1.
[0011] In any embodiment of the therapeutic composition, the physical trauma may be a spinal cord injury (SCI), traumatic brain injury (TBI), or both. In any embodiment of the therapeutic composition, the physical trauma may be an SCI. [0012] In any embodiment of the therapeutic composition, the composition may further comprise an absorption enhancing agent, adjuvant, buffer, excipient, pharmaceutical carrier, preservative, stabilizer, or any combination thereof.
[0013] In another aspect, the present disclosure provides a method of treatment of a traumatic injury in mammalian subject in need thereof, the method comprising: administering to the subject a clinically effective amount of the composition of any of the preceding paragraphs. In any embodiment of the method of treatment, the traumatic injury may be an SCI, TBI, or both. In any embodiment, a therapeutically effective dose is a dose that results in mitigation of clinical effects of neurotraumatic secondary injury. In any embodiment, the mammalian subject may be a human. In any embodiment, the composition may be administered orally, parenterally, subcutaneously, intramuscularly, intravenously, intraarterially, intradermally, intrathecally, epidurally, transdermally, rectally, nasally, topically, buccally, sublingually, vaginally, intraperitoneally, intrapulmonarily, intranasally, intraocularly, or any combination thereof. In any embodiment, the therapeutically effective dose may be administered within 24 hours of the mammalian subject having sustained a primary injury.
[0014] In still another aspect, the present disclosure provides a method of manufacturing an anti-CDld antibody or antibody fragment, the method comprising: producing the antibody or antibody fragment in cell culture or hybridoma; and purifying the antibody or antibody fragment. [0015] In yet another aspect, the present disclosure provides a method of increasing the concentration of tissue-reparing monocytes and/or tissue-repairing macrophages in the local tissue microenvironment of a neurotraumatic primary injury, in a mammalian subject in need thereof, the method comprising administering a therapeutically effective amount of an inhibitor of CD Id to the subject after the occurrence of the primary injury. In any embodiment, the inhibitor of CDld may comprise a therapeutic composition as described in the preceding paragraphs, including, e.g., an antibody and/or antibody fragment. In any embodiment, the primary injury may be result of mechanical force causing traumatic brain injury or spinal cord injury or both. In any embodiment, the mammalian subject may be a human. In any embodiment, the therapeutically effective dose is administered within 24 hours of the mammalian subject having sustained the primary injury. In any embodiment, the therapeutically effective dose is administered orally, parenterally, subcutaneously, intramuscularly, intravenously, intraarterially, intradermally, intrathecally, epidurally, transdermally, rectally, nasally, topically, buccally, sublingually, vaginally, intraperitoneally, intrapulmonarily, intranasally, intraocularly, or any combination thereof.
[0016] In still yet another aspect, the present disclosure provides a system for treatment of physical trauma, the system comprising a therapeutic composition substantially as described in the preceding paragraphs, the composition pre-loaded into a hypodermic needle. In any embodiment, the hypodermic needle may comprise an autoinjector.
BRIEF DESCRIPTION OF THE DRAWINGS
[0017] Fig. 1 depicts a series of fluorescence micrographs. Left panel: IFM analysis of CD Id (red) plus either NeuN (neurons, green), GFAP (astrocytes, green), Ibal (microglia, green), Oligo2 (oligodendrocytes, green), or CD31 (endothelial cells, green) in primary rat spinal cord cells. Hoechst dye (Hst, blue) was for nuclear staining. Right panel: Immunohistochemistry (IHC) analysis of CDld expression in normal rat spinal cord tissue. Cells CDld+ only or CDld+Ibal+, CDld'Olig2', or CDldlCD31l double positive (white arrowheads); cells not co-labeled with CDld/NeuN or CDld/GFAP (white arrows). White bar: 50 pM.
[0018] Fig. 2 is a diagram of an experimental protocol. Spinal cord from days 1, 3 and 7 post-SCI vs sham-injured rats.
[0019] Fig. 3 depicts pooled data showing CDld expression in the injured epicenter, as well rostral and caudal to the injury site over the 7-day analysis post-SCI. Student’s /-test: *** p < 0.001. n > 3 per group. S, sham.
[0020] Fig. 4 shows CDld in the injured epicenter and 1.25 mm away (rostral and caudal) 7-days post-SCI. White short and long bars: 80 pM and 300 pM, respectively.
[0021] Fig. 5 is a qRT-PCR analysis of wild-type (WT) mouse spinal cord CDld mRNA levels over 7-days post-SCI.
[0022] Fig. 6 depicts pooled data of lesion area size and spared white matter (SWM) from WT (blue bars) and CDldKO (red bars) mice.
[0023] Fig. 7 is representative micrograph images of spinal cord Nissl (upper panel) and Luxol Fast Blue (LFB) staining (lower panel) showing lesion area size and SWM, respectively.
[0024] Figs. 8A-8B are 3-dimensional reconstructions of the injured spinal cord areas based on Nissl and LFB staining data from WT (Fig. 8A) and CDldKO (Fig. 8B) mice. [0025] Fig. 9 is representative images of THC analysis of viable spinal cord motor neurons (red arrows) in CDldKO vs WT mice post-SCI using Nissl staining.
[0026] Figs. 10A-10C are graphs depicting pooled data of 3D reconstruction results (blue bars: WT mice; red bars: CDldKO mice).
[0027] Fig. 11 depicts pooled data of viable spinal cord motor neurons in CDldKO (red bars) vs WT (blue bars) mice post-SCI from Nissl staining. Student’s t-test: *p<0.05; ns, not significant. WT-SCI, n=12; CDldKO-SCI, n=13; WT-sham, n=6; CDldKO-sham, n=6.
[0028] Fig. 12 depicts a timeline of an experimental design and timepoints post-SCI for the BMS, Grid-Walking and Rotarod tests to evaluate locomotion deficits, sensorimotor deficits, and motor coordination.
[0029] Fig. 13 is a series of cartoon illustrations highlighting an experimental design, including the Basso Mouse Scale (BMS), Grid-Walking and Rotarod tests to evaluate locomotion deficits, sensorimotor deficits, and motor coordination.
[0030] Fig. 14 depicts pooled data showing BMS scores on day 28 post-SCI.
[0031] Figs. 15A-15B are depictions of pooled data showing foot drop errors (Fig. 15 A) and latency (Fig. 15B, 30 rpm) from WT and CDldKO mice on day 28 post-SCI.
[0032] Fig. 16 is a cartoon illustration of a transcranial magnetic motor-evoked potentials (tcMMEP) setup, designed to assess the functional integrity of the spinal cord’s descending axonal pathways following a SCI.
[0033] Figs. 17A-17C depict pooled data of tcMMEP showing the evoked potentials (Fig. 17A), total response rate (Fig. 17B), and potential amplitude (Fig. 17C) from WT and CDldKO mice on day 28 post-SCI.
[0034] Figs. 18A-18D are Representative recordings of tcMMEP showing the potential amplitude, latency and response rate in CDldKO or WT mice on day 28 post-SCI or sham injury. Statistical analyses: two-way analysis-of-variance (ANOVA) (BMS), %2 test (response), or a oneway ANOVA for all other groups. *p<0.05 **p<0.01 ***£><0.001; ns, not significant. WT-SCI, n=12; CDldKO-SCI, n=13; WT-sham, n=6; CDldKO-sham, n=6.
[0035] Fig. 19 depicts a series of fluorescence micrographs of injured spinal cord tissues from WT and CDldKO mice, including the injured epicenter and 0.5-1.0 mm both rostral and caudal away on day 7 post-SCI. [0036] Figs. 20A-20C are depictions of data of numbers of cells per mm3 for BrdU+ cells (Fig. 20A), Ibal+ cells (Fig. 20B), and BrdU+Ibal+ cells (Fig. 20C) in the injured epicenter and 0.5-1.0 mm rostral and caudal away. Student’s /-test was used for statistical analysis. *p<0.05, ** ?<0.01, ***p<0.001. n=3/group. Epi, Epicenter. Hst, Hoechst dye (blue) for DNA staining.
[0037] Fig. 21 is a series of graphs depicting gating strategy for flow cytometric analysis of immune cells in the spinal cord (upper panel) and liver (lower panel) of WT versus CDldKO mice post-SCI. The gated M/M<|) population (M) showing the % of Ly-6CHlgh versus Ly- 6cLo«F4/3oHigh M/M(|) in the spinal cord and liver. At the indicated timepoints post-SCI, whole liver and 2 mm of spinal cord tissue including the injured epicenter and 1 mm rostral and caudal away were harvested. Single-cell suspensions were stained with Zombie Violet™ fixable viability dye (FVD) to exclude FVD-positive dead cells, anti-CD16/CD32 mAbs for blocking FcR binding and fluorochrome-conjugated mAbs against mouse CD45, CD3, CD4, CD8, CD1 lb, Ly-6G, Ly- 6C, F4/80, B220, and NK1.1. Tissue-infiltrating leukocytes (LYM) were defined as CD45Hlgh cells. Within the CD45Hlgh population, polymorphonuclear neutrophils (PMNs) were identified by Ly-6G expression, T cells as CD45HighCD3+ cells, CD4 T cells as CD45+CD3+CD4+, CD8 T cells as CD45+CD3 CD8+, B cells as CD45+B220+, NKT cells (CD45+CD3+NK1.1+) and monocytes/macrophages (M/M$) as CD45 CDl lb Ly-6G . M/M(j> were further divided into inflammatory Ly-6CHlgh and tissue-repairing Ly-6CLowF4/80Hlgh subsets.
[0038] Figs. 22A-22B are depictions of pooled data of Ly-6CHlgh versus Ly- 6CLowF4/80High M/M(|) in the spinal cord (Fig. 22A) and liver (Fig. 22B) on day 1 (n=4/group), day 3 (n=4/group), day 7 (n=9/group) and day 28 (n=4/group) post-SCI.
[0039] Fig. 23 is a depiction of pooled data showing Ly-6CHlgh versus Ly-6CLowF4/80Hlgh M/Mcj) in the spinal cords of WT and CDldKO mice on day 7 post-SCI (n=9/group).
[0040] Fig. 24 is a series of of pooled data from the multiplex immunoassay showing the tissue levels of eight proinflammatory/anti-inflammatory mediators that were significantly different between groups of WT and CDldKO mice. Student’s /-test was used for statistical analysis. *p<0.05, ** i<0.01. n = >4 per group.
[0041] Fig. 25 illustrates layout and timeline for pre-injury training, SCI, a- galactosylceramide (a-GalCer) administration via intraperitoneal injection and, motor and behavioral assessments of WT mice.
[0042] Fig. 26 is a depiction of pooled data of BMS scores. [0043] Figs. 27A-27B are depictions of pooled data of Grid-Walking (Fig. 27 A), and Rotarod tests (Fig. 27B)
[0044] Fig. 28 is a layout and timeline for pre-injury training, SCI and, motor and behavioral assessments ofWT and Traj l8KO (Type I NKT cell-deficient) mice.
[0045] Fig. 29 is a depiction of pooled data of BMS in WT and Traj 18KO mice.
[0046] Figs. 30A-30C are depictions of Grid-Walking (Fig. 30A), Rotarod (Fig. 30B), and tcMMEP (Fig. 30C) tests. A two-way ANOVA was used for BMS data analysis. Student’s /-test was used for analyzing Grid-Walking and Rotarod test results. *p<0.05. WT-SCI, n=6; Traj 18KO- SCI, n=7; sham + PBS, n=6; sham + a-Gal, n=6; SCI + PBS, n=7; SCI + a-Gal, n=8. a-Gal: a- GalCer; non-res: non-response.
[0047] Fig. 31 is a layout and timeline for pre-injury training, SCI, anti-mouse CD Id mAh administration via intraperotineal injection, spinal cord tissue collection and, motor and behavioral assessments of WT and CDldKO mice. Anti-mouse CDld (1H6) or an isotype control mAb was administered intraperotineally (50 pg/mouse) into WT mice at various times post-SCI.
[0048] Fig. 32 is a depiction of pooled BMS data.
[0049] Figs. 33 A-33B are depictions of pooled Grid-Walking (Fig. 33A) and Rotarod tests
(Fig. 33B) data.
[0050] Fig. 34 is a series of representative micrograph images of Nissl (upper panel) and Luxol fast blue (LFB) staining (lower panel) on histological changes of spinal cord tissue lesion size and spared white matter (SWM).
[0051] Figs. 35A-35B are depictions of pooled data showing spinal cord tissue lesion size (Fig. 35 A) and spared white matter volume (Fig. 35B) in SCI mice treated with the anti-CDld mAh or isotype control mAb. Two-way ANOVA was used for analyzing the BMS data. Student’s /-test was used for analyzing foot drop errors, latency, lesion area and amount of spared white matter (SWM). */?<0.05, **/?<0.01, *** /?<0.001. CDld mAb + WT-SCI, n=14; isotype mAb + WT-SCI, n=12.
[0052] Fig. 36 is a depiction of FACS gating strategy for flow cytometric analysis of immune cells in spinal cord (upper panel) and liver (lower panel) of WT versus CDldKO mice post-SCI. Gated M/M(|) (M) showing the % of Ly-6CHlgh versus Ly-6CLowF4/80Hlgh M/M(|) in the spinal cord and liver. NKT cells (CD45+CD3+NK1.1+) were barely detected in the spinal cord from both WT and CDldKO mice with and without a SCI (right panel). After SCI, mice were perfused with heparinized PBS and then whole liver and 3 mm spinal cord tissues including the injured epicenter and 1.5 mm away from the injured epicenter on both the rostral and caudal sides were harvested from each mouse at various timepoints. The liver and spinal cord tissues were then processed into single-cell suspensions. Cells were stained with Zombie Violet™ FVD to exclude FVD-positive dead cells, followed by incubation with CD16/CD32 mAbs for blocking FcR binding, and fluorochrome-conjugated mAbs against mouse CD45, CD3, CD4, CD8, CD1 lb, Ly- 6G, Ly-6C, F4/80, B220, and NK1.1. Appropriate isotype controls were used at the same protein concentrations as the test mAbs. Tissue-infiltrating leukocytes (LYM) were defined as CD45Hlgh cells. Within the CD45Hlgh population, polymorphonuclear neutrophils (PMNs) were identified by Ly-6G expression, T cells as CD45HighCD3+ cells, CD4 T cells as CD45+CD3+CD4+, CD8 T cells as CD45 CD3+CD8+, B cells as CD45+B220+, NKT cells (CD45+CD3+NK1.1+), and monocytes/macrophages (M/M<j>) as CD45+CD1 l b Ly-6G . M/M<[) were further divided into inflammatory (Ly-6CHlgh) and tissue-repairing (Ly-6CLowF4/80Hlgh) subsets.
[0053] Fig. 37 is a depiction of a data series of pooled data of CD45+ lymphocytes total T cells, CD4+ T cells, CD8+ T cells, B220 B cells, and PMN in the spinal cord on days 1 (n=4/group), 3 (n=4/group), 7 (n=4/group) and 28 (n=4/group) post-SCI.
[0054] Fig. 38 is a uniform manifold approximation and projection (UMAP) plot showing 12 major cell types, pooled from each group of mice. Each dot represents a single cell. WT and CDldKO mice received either a SCI or sham injury. On day 7, a 2-centimeter section of spinal cord containing the injured site was used for isolating single-cell populations for single-cell RNA sequencing (scRNA-seq) analysis.
[0055] Fig. 39 is a dot-size graphical illustration of gene expression of Vegfa and Nr4al in different cell types in spinal cord tissues including microvascular endothelial cells (ECs), neurons, astrocytes, oligodendrocytes (ODCs), precursor oligs, microglia, and macrophages (Ml and M2). [0056] Fig. 40 is a dot-size graphical illustration of transcriptional signature of microglia activating. (Clustering analysis was performed using RStudio®, version 3.6.1.) Four mice were used per condition.
[0057] Fig. 41 is an exemplary 3D crystal structure model of human CD Id protein.
[0058] It should be understood that the figures are exemplary in nature and in no way serve to limit the scope of the invention. DETAILED DESCRIPTION OE THE INVENTION
[0059] As shown by this detailed description, the present disclosure contemplates multiple and various embodiments, including, without limitation, the following example embodiments.
[0060] While example embodiments of the disclosure have been illustrated and described in detail in the drawings and foregoing description, the same is to be considered as illustrative and not restrictive in character, it being understood that only certain example embodiments have been shown and described and that all changes and modifications that come within the spirit of the claimed inventions are desired to be protected. It should be understood that while the use of words such as preferable, preferably, preferred or more preferred utilized in the description above indicates that the feature so described may be more desirable, it nonetheless may not be necessary and embodiments lacking the same may be contemplated as within the scope of the invention, the scope being defined by the claims that follow. In reading the claims, it is intended that when words such as “a,” “an,” “at least one,” or “at least one portion” are used there is no intention to limit the claim to only one item unless specifically stated to the contrary in the claim. When the language “at least a portion” and/or “a portion” is used the item can include a portion and/or the entire item unless specifically stated to the contrary.
[0061] “Antibody fragments” comprise a portion of an intact antibody, in an embodiment, comprising the antigen-binding region thereof. Examples of antibody fragments include Fab, Fab', F(ab')2, and Fv fragments; diabodies; linear antibodies; single-chain antibody molecules; and multispecific antibodies formed from antibody fragments. Papain digestion of antibodies produces two identical antigen-binding fragments, called “Fab” fragments, each with a single antigenbinding site, and a residual “Fc” fragment, whose name reflects its ability to crystallize readily. Pepsin treatment yields an F(ab')2 fragment that has two antigen-combining sites and is still capable of cross-linking antigen. “Fv” is the minimum antibody fragment which contains a complete antigen-binding site. Preferably, a two-chain Fv species consists of a dimer of one heavy - and one light-chain variable domain in tight, non-covalent association. In a single-chain Fv (scFv) species, one heavy- and one light-chain variable domain can be covalently linked by a flexible peptide linker such that the light and heavy chains can associate in a “dimeric” structure analogous to that in a two-chain Fv species. It is in this configuration that the three hypervariable regions (HVRs, also referred to as complementarity determining regions (CDRs)) of each variable domain interact to define an antigen-binding site. Collectively, the six HVRs confer antigen-binding specificity to the antibody. However, even a single variable domain (or half of an Fv comprising only three HVRs specific for an antigen) has the ability to recognize and bind antigen, although at a lower affinity than the entire binding site. The term “diabodies” refers to antibody fragments with two antigen-binding sites, which fragments comprise a heavy-chain variable domain (VH) connected to a light-chain variable domain (VL) in the same polypeptide chain (VH-VL). By using a linker that is too short to allow pairing between the two domains on the same chain, the domains are forced to pair with the complementary domains of another chain and create two antigen-binding sites. Diabodies may be bivalent or bispecific. Diabodies are described more fully in, for example, EP 0 404 097; WO 1993/01161; Hudson et al., Nat. Med. 9 (2003) 129-134; and Hollinger et al., PNAS USA 90 (1993) 6444-6448. Triabodies and tetrabodies are also described in Hudson et al., Nat. Med. 9 (2003) 129-134.
[0062] The term “monoclonal antibody” as used herein refers to an antibody obtained from a population of essentially homogeneous antibodies, i.e., the individual antibodies comprising the population are identical except for possible mutations, e.g., naturally occurring mutations, that may be present in minor amounts. Thus, the modifier “monoclonal” indicates the character of the antibody as not being a mixture of discrete antibodies. Preferably, such a monoclonal antibody includes an antibody comprising a polypeptide sequence that binds a norovirus polypeptide, wherein the target-binding polypeptide sequence was obtained by a process that includes the selection of a single binding polypeptide sequence from a plurality of polypeptide sequences. For example, the selection process can be the selection of a unique clone from a plurality of clones, such as a pool of hybridoma clones, phage clones, or recombinant DNA clones.
[0063] The term “single-domain antibody” (VHH) or “nanobody”, relates to an antibody fragment comprising one variable antibody domain and is, in principle, known to the skilled person. A review is provided, e.g., in Muyldermanns et al. (2009), Vet Immunol Immunopathol. 128(1—3): 178. The VHH may comprise the CDRs of a heavy-chain antibody, preferably obtained from a camelid (alpaca, dromedar, camel, llama) or shark immunized with a target polypeptide. The VHH may have the binding properties as specified above. It is understood by the skilled person that the binding properties of an antibody, in particular of a VHH, usually are conserved if amino acids, in particular those not comprised in a CDR, are exchanged. Accordingly, the term binding polypeptide also relates to polypeptides comprising an amino acid sequence having at least 70%, more preferably at least 80%, still more preferably at least 90%, even more preferably at least 95%, most preferably at least 99%, sequence identity to the CDR.
[0064] The present disclosure relates to systems, materials, and methods for treatment of traumatic injury in a mammalian subject in need thereof. The present disclosure further relates to improvements in therapeutic discovery processes, particularly for developing therapeutics that target CD Id. Such therapeutic may aid in reducing the clinical effects of secondary injury following a mechanical trauma, including a neurotrauma, and may aid in improving tissue healing and recovery. The cell surface molecule, CDld, is known to be recognized by cells of the immune system. To the inventors’ knowledge, the present disclosure represents the first observation that the CDld molecule significantly contributes to neuroinflammation following a neurotrauma in a manner independent of the CDld/iNKT cell axis. This is important, because this invention reveals CDld as a novel and surprising target following a neurotrauma such as an acute spinal cord injury, for which there are currently no safe and effective treatments.
[0065] Traumatic spinal cord injury (SCI) represents a significant public health issue, affecting more than 2.5 million people worldwide with approximately 130,000 new injuries reported annually. SCI is a common cause of permanent disability in children and adults with a tremendous socioeconomic impact on affected individuals and the health care system. SCI, like other forms of neurotrauma such as traumatic brain injury (TBI), is composed of primary and secondary injuries. A primary injury is caused by the initial traumatic impact, whereas the secondary injury is an indirect result of destructive immunological, inflammatory, neurotoxic, and biochemical cascades that are initially triggered by the primary injury.
[0066] Secondary injury remains an important determinant of SCI outcome as its’ damage can often be in excess of that caused by primary injury. Secondary injury begins within minutes following the initial primary injury and can be temporally divided into acute (<48 hours after the traumatic event), sub-acute (48 hours up to 14 days), intermediate (14 days to 6 months), and chronic (> 6 months) phases.
[0067] In the clinical management of SCI, neurological outcomes are generally determined at 72 hours and less after SCI, indicating that acute and subacute phases represent the most important time window in which to achieve optimal tissue repair and neurological recovery. Accordingly, neuroimmune and neuroinflammatory responses play a central role in orchestrating the secondary injury mechanisms in the acute and sub-acute phases of SCI. During the acute and subacute phases, immune cells such as monocytes/macrophages (M/M<[)), T cells, B cells, and neutrophils infiltrate the spinal cord to interact with neural cells and produce inflammatory cytokines, chemokines, and neurotoxic molecules that contribute to cell death and tissue degeneration. Although many immune cell types are involved in these early phases of SCI, because of their highly flexible programming, M/M<j) have been shown to exhibit critical regulatory activity in response to environmental cues and play an active and dual role in SCI. Whereas M/M$ responses are characterized by the accumulation of inflammatory M/M^ in the acute phase to clear cellular debris, there is also the production of inflammatory cytokines/chemokines such as IL-ip, IL-6, IL-8, and TNF-a, thereby exerting both beneficial and detrimental effects. Inflammatory M/M responses are tightly regulated in a timely manner.
[0068] Monocytes/macrophages (M/M([)) may be subdivided into “inflammatory” subtype, which may be characterized by expression markers as Ly-6CHlgh, and “tissue-repair” subtype, which may be characterized by expression markers as Ly-6CLowF4/80Hlgh. See, e.g., Sun Y, et al. Review: Macrophage phenotype in Liver Injury and Repair, Scandinavian J Immunol. 2017;85: 166-174; Wynn T & Vannell K, Macrophages in tissue repair, regeneration, and fibrosis, Immunity 2016;44(3): 450-462. Importantly, inflammatory M/M<[) can be polarized into tissue-repairing M/M(|) by cytokines such as IL-4 in the local tissue microenvironment. These then produce growth factors such as vascular endothelial growth factor (VEGF) and anti-inflammatory mediators (e.g., IL-4, IL-10, and TGF-pi) to foster inflammation resolution, tissue repair and wound healing. In addition, M/M$ and microglia (tissue-resident macrophages that are exclusively derived from yolk sac-derived progenitors) coordinate cellular interactions with neuronal and non-neuronal cells in the spinal cord to restore tissue homeostasis after SCI. However, prior to the present disclosure the cellular and molecular mechanisms of immune homeostasis and resolution of inflammation in the spinal cord after SCI were not understood. It is critical to unravel these mechanisms for developing effective treatments for this devastating condition to fill a significant unmet medical need.
[0069] A neuotrauma such as an SCI leads to tissue destruction and cellular damage which, in turn, rapidly release endogenous “danger” molecules, known as damage-associated molecular patterns (DAMPs). DAMPs prime innate immune cells such as infiltrating M/M([) and neutrophils, as well as the resident microglia and astrocytes in the central nervous system (CNS) to induce sterile neuroimmune/neuroinflammatory responses. Because lipids are essential structural and functional components of all cell types, lipids are also released and have been identified as DAMPs. The CNS has a rich lipid composition with about 75% of all mammalian lipid species that are exclusively present in neural tissues, demonstrating the unique requirements of lipids for neural cell functions such as synaptogenesis and neurogenesis, impulse transmission, and the development and maintenance of the CNS. The glycolipids represent a dominant class of lipids in the myelin bilayer of neural cells, which may act as DAMPs to directly trigger innate immune responses in the spinal cord after SCI. The released lipids following a neurotrauma may also be processed as lipid antigens that are presented by CD Id molecules to natural killer T (NKT) cells, a subset of CD Id-restricted T cells that bridges innate and adaptive immunity.
[0070] Lipid species have in general been known for many years to have immunogenic effects. See V. Sriram, et al., Cell wall glycosphingolipids of Sphingomonas paucimobilis are CDld- specific ligands for NKT cells. Eur J Imm unol 35, 1692-1701 (2005); see also, e.g., Razin, S, et al., Immunogenicity of Mycoplasma pneumonia Glycolipids: A Novel Approch to the Production of Antisera to Membrane Lipids, Proc Nat ’I Acad Sci USA 1970 Oct; 67(2): 590-597. The cellsurface protein CD Id (Fig. 41) is a major histocompatibility complex (MHC) class Llike molecule that, unlike the classical MHC class I molecules that present peptides to CD8 T cells, presents lipid antigens to NKT cells. The CDld/NKT cell axis has been shown to be important in a variety of diseases including some CNS diseases such as multiple sclerosis (MS) and Experimental Allergic Encephalomyelitis (EAE). In addition to presentation of lipid antigens to NKT cells, CDld also exerts intrinsic signaling to regulate immune and inflammatory responses. Neither CDld nor NKT cells have been studied in the context of neurotrauma.
[0071] The responses of CDld knockout mice (CDldKO, which do not have NKT cells) to a contusive SCI at the 10th thoracic vertebra (T10) were analyzed. Using a diverse array of motor assessments and behavioral assessments, it was discovered that CDldKO mice recovered from neurotrauma significantly faster from SCI than wildtype (WT) mice. Concomitantly, less damage to the spinal cord and more spared white matter were observed in CDldKO mice versus WT mice. In addition, the levels of inflammatory cytokines and activation/proliferation of microglia/ M<[) were dramatically reduced, whereas anti-inflammatory cytokines (e.g., IL-4) and growth factors (e.g., VEGF) were detected at much higher amounts in spinal cord tissues of CDldKO mice when compared to WT mice. In the subacute phase of SCI (i.e., day 7 post-SCI), CDldKO spinal cord tissues had a significantly higher frequency of tissue-repairing M/M([> versus WT mice. [0072] Treatment of SCI mice with a CDld-specific antibody significantly enhanced neuroprotection and improved functional recovery. Strikingly, these CDld effects appear to be mediated by mechanisms independent of the canonical CDld/NKT cell axis. Thus, CDld promotes neuroimmune/neuroinflammatory responses following SCI and represents a therapeutic target for neurotraumas such as SCI.
[0073] Provided in the present disclosure are systems, materials, and methods for treatment of traumatic injury in a mammalian subject in need thereof. The present disclosure further relates to improvements in therapeutic discovery processes, particularly for developing therapeutics that target CDld.
[0074] In an aspect, the present disclosure provides a therapeutic composition for treatment of physical trauma, the therapeutic comprising an inhibitor of CDld. In some embodiments, the composition may comprise a small-molecule pharmaceutical. In some embodiments, the composition may comprise an antibody or antibody fragment. In such embodiments, the antibody or antibody fragment may comprise any of a F(ab')2, F(ab')2 fragment, Fab', F(ab)2, Fab, Fab fragment, Fv, sFv, single-chain variable fragment (scFv), single-domain antibody (sdAb), nanobody, diabody, triabody, minibody, or combination thereof. The antibody or antibody fragment may specifically bind to a CDld protein or peptide having 70% or greater sequence identity to SEQ ID NO: 1. The antibody or antibody fragment may specifically bind to a CDld protein or peptide having at least 70%, at least 75%, at least 80%, at least 85%, at least 90%, at least 95%, at least 99% or 100% sequence identity to SEQ ID NO: 1.
[0075] In any embodiment of the therapeutic composition, the physical trauma may be a spinal cord injury (SCI), traumatic brain injury (TBI), or both. In any embodiment of the therapeutic composition, the physical trauma may be an SCI.
[0076] In any embodiment of the therapeutic composition, the composition may further comprise an absorption enhancing agent, adjuvant, buffer, excipient, pharmaceutical carrier, preservative, stabilizer, or any combination thereof.
[0077] In another aspect, the present disclosure provides a method of treatment of a traumatic injury in mammalian subject in need thereof, the method comprising: administering to the subject a clinically effective amount of the composition of any of the preceding paragraphs. In any embodiment of the method of treatment, the traumatic injury may be an SCI, TBI, or both. In any embodiment, a therapeutically effective dose is a dose that results in mitigation of clinical effects of neurotraumatic secondary injury. In any embodiment, the mammalian subject may be a human. In any embodiment, the composition may be administered orally, parenterally, subcutaneously, intramuscularly, intravenously, intraarterially, intradermally, intrathecally, epidurally, transdermally, rectally, nasally, topically, buccally, sublingually, vaginally, intraperitoneally, intrapulmonarily, intranasally, intraocularly, or any combination thereof. In any embodiment, the therapeutically effective dose may be administered within 24 hours of the mammalian subject having sustained a primary injury.
[0078] In still another aspect, the present disclosure provides a method of manufacturing an anti-CDld antibody or antibody fragment, the method comprising: producing the antibody or antibody fragment in cell culture or hybridoma; and purifying the antibody or antibody fragment. [0079] In yet another aspect, the present disclosure provides a method of increasing the concentration of tissue-reparing monocytes and/or tissue-repairing macrophages in the local tissue microenvironment of a neurotraumatic primary injury, in a mammalian subject in need thereof, the method comprising administering a therapeutically effective amount of an inhibitor of CD Id to the subject after the occurrence of the primary injury. In any embodiment, the inhibitor of CD Id may comprise a therapeutic composition as described in the preceding paragraphs, including, e.g., an antibody and/or antibody fragment. In any embodiment, the primary injury may be result of mechanical force causing traumatic brain injury or spinal cord injury or both. In any embodiment, the mammalian subject may be a human. In any embodiment, the therapeutically effective dose is administered within 72 hours of the mammalian subject having sustained the primary injury. In any embodiment, the therapeutically effective dose is administered within 24 hours of the mammalian subject having sustained the primary injury. In any embodiment, the therapeutically effective dose is administered orally, parenterally, subcutaneously, intramuscularly, intravenously, intraarterially, intradermally, intrathecally, epidurally, transdermally, rectally, nasally, topically, buccally, sublingually, vaginally, intraperitoneally, intrapulmonarily, intranasally, intraocularly, or any combination thereof.
[0080] In still yet another aspect, the present disclosure provides a system for treatment of physical trauma, the system comprising a therapeutic composition substantially as described in the preceding paragraphs, the composition pre-loaded into a hypodermic needle. In any embodiment, the hypodermic needle may comprise an autoinjector. [0081] The scope of the present disclosure may be further understood by way of reference to the following non-limiting clauses:
[0082] Clause 1. A therapeutic composition for treatment of physical trauma, the therapeutic comprising an inhibitor of CD Id.
[0083] Clause 2. The composition of clause 1, wherein the inhibitor comprises a small-molecule pharmaceutical.
[0084] Clause 3. The composition of clause 1, wherein the inhibitor comprises an antibody or antibody fragment.
[0085] Clause 4. The composition of clause 3, wherein the antibody or antibody fragment comprise any of a F(ab')2, F(ab')2 fragment, Fab', F(ab)2, Fab, Fab fragment, Fv, sFv, single-chain variable fragment (scFv), single-domain antibody (sdAb), nanobody, diabody, triabody, minibody, or combination thereof.
[0086] Clause 5. The composition of clause 3 or 4, wherein the antibody or antibody fragment specifically binds a CD Id protein having at least 70% amino acid sequence identity to SEQ ID NO: 1.
[0087] Clause 6. The composition of clause 3 or 4, wherein the antibody or antibody fragment specifically binds a CD Id protein having at least 80% amino acid sequence identity to SEQ ID NO: 1.
[0088] Clause 7. The composition of clause 3 or 4, wherein the antibody or antibody fragment specifically binds a CD Id protein having at least 90% amino acid sequence identity to SEQ ID NO: 1.
[0089] Clause 8. The composition of clause 3 or 4, wherein the antibody or antibody fragment specifically binds a CD Id protein having at least 95% amino acid sequence identity to SEQ ID NO: 1.
[0090] Clause 9. The composition of any of clauses 1-8, wherein the physical trauma is a spinal cord injury or traumatic brain injury or both.
[0091] Clause 10. The composition of any of clauses 1-8, wherein the physical trauma is a spinal cord injury.
[0092] Clause 11. The composition of any of clauses 1-10, further comprising an absorption enhancing agent, adjuvant, buffer, excipient, pharmaceutical carrier, preservative, stabilizer, or any combination thereof. [0093] Clause 12. A method of treatment of a traumatic injury in mammalian subject in need thereof, the method comprising: administering to the subject a clinically effective amount of the composition of any of clauses 1-11.
[0094] Clause 13. The method of clause 12, wherein the traumatic injury is atraumatic brain injury or spinal cord injury or both.
[0095] Clause 14. The method of 13, wherein a therapeutically effective dose is a dose that results in mitigation of clinical effects of neurotraumatic secondary injury.
[0096] Clause 15. The method of any of clauses 12-14, wherein the mammalian subject is a human.
[0097] Clause 16. The method of any of clauses 12-15, wherein the composition is administered orally, parenterally, subcutaneously, intramuscularly, intravenously, intraarterially, intradermally, intrathecally, epidurally, transdermally, rectally, nasally, topically, buccally, sublingually, vaginally, intraperitoneally, intrapulmonarily, intranasally, intraocularly, or any combination thereof.
[0098] Clause 17. The method of any of clauses 12-16, wherein the therapeutically effective dose is administered within 24 hours of the mammalian subject having sustained a primary injury.
[0099] Clause 18. The method of any of clauses 12-16, wherein the therapeutically effective dose is administered within one hour of the mammalian subject having sustained a primary injury.
[00100] Clause 19. The method of any of clauses 12-16, wherein the therapeutically effective dose is administered within 10 minutes of the mammalian subject having sustained a primary injury.
[00101] Clause 20. A method of manufacturing the composition of any of clauses 3-11, the method comprising: producing the antibody or antibody fragment in cell culture or hybridoma; and purifying the antibody or antibody fragment.
[00102] Clause 21. A method of increasing the concentration of tissue-reparing monocytes and/or tissue-repairing macrophages in the local tissue microenvironment of a neurotraumatic primary injury, in a mammalian subject in need thereof, the method comprising administering a therapeutically effective amount of the composition of any of clauses 3-11 to the subject after the occurrence of the primary injury. [00103] Clause 22. The method of clause 20, wherein the neurotaumatic primary injury is a result of mechanical force causing traumatic brain injury or spinal cord injury or both.
[00104] Clause 23. The method of clauses 20 or 21, wherein the mammalian subject is a human.
[00105] Clause 24. The method of any of clauses 20-22, wherein the therapeutically effective dose is administered within 24 hours of the mammalian subject having sustained the primary injury.
[00106] Clause 25. The method of any of clauses 20-23, wherein the therapeutically effective dose is administered orally, parenterally, subcutaneously, intramuscularly, intravenously, intraarterially, intradermally, intrathecally, epidurally, transdermally, rectally, nasally, topically, buccally, sublingually, vaginally, intraperitoneally, intrapulmonarily, intranasally, intraocularly, or any combination thereof.
[00107] Clause 26. A method of treating a traumatic injury in mammalian subject in need thereof, the method comprising: administering to the subject a clinically effective amount of anti-CDld antibody or antibody fragment.
[00108] Clause 27. The method of treating a traumatic injury of clause 26, wherein the anti-CDld antibody comprises an IgG2b.
[00109] Clause 28. The method of treating a traumatic injury of clause 26 or 27, wherein the anti-CDld antibody comprises mAb 1H6.
[00110] Clause 29. The method of treating a traumatic injury of any of clauses 26-28, wherein the traumatic injury is a traumatic brain injury or spinal cord injury or both.
[00111] Clause 30. The method of treating a traumatic injury of any of clauses 26-29, wherein a therapeutically effective dose is a dose that results in mitigation of clinical effects of neurotraumatic secondary injury.
[00112] Clause 31. The method of treating a traumatic injury of any of clauses 26-30, wherein the mammalian subject is a human.
[00113] Clause 32. The method of treating a traumatic injury of any of clauses 26-31, wherein the composition is administered orally, parenterally, subcutaneously, intramuscularly, intravenously, intraarterially, intradermally, intrathecally, epidurally, transdermally, rectally, nasally, topically, buccally, sublingually, vaginally, intraperitoneally, intrapulmonarily, intranasally, intraocularly, or any combination thereof. [00114] Clause 33. The method of treating a traumatic injury of any of clauses 26-32, wherein the therapeutically effective dose is administered within 24 hours of the mammalian subject having sustained a primary injury.
[00115] Clause 34. The method of treating a traumatic injury of any of clauses 26-33, wherein the therapeutically effective dose is administered within one hour of the mammalian subject having sustained a primary injury.
[00116] Clause 35. The method of treating a traumatic injury of any of clauses 26-34, wherein the therapeutically effective dose is administered within 10 minutes of the mammalian subject having sustained a primary injury.
[00117] Clause 36. A method of increasing the concentration over baseline concentration of tissue-reparing monocytes and/or tissue-repairing macrophages in the local tissue microenvironment of a current or future neurotraumatic primary injury, in a mammalian subject in need thereof, the method comprising administering a therapeutically effective amount of antiCD Id antibody or antibody fragment to the subject.
[00118] Clause 37. The method of clause 36, wherein the administration is before the occurrence of the neurotraumatic primary injury.
[00119] Clause 38. The method of clause 36, wherein the administration is after the occurrence of the neurotraumatic primary injury.
[00120] Clause 39. The method of clause 38, wherein the administration is within 24 hours after the occurrence of the neurotraumatic primary injury.
[00121] Clause 40. The method of clause 38, wherein the administration is within one hour after the occurrence of the neurotraumatic primary injury.
[00122] Clause 41. The method of clause 38, wherein the administration is within ten minutes after the occurrence of the neurotraumatic primary injury.
[00123] Clause 42. A system for treatment of physical trauma, the system comprising the composition of any of clauses 1-11 pre-loaded into a hypodermic needle.
[00124] Clause 43. A system for treatment of physical trauma, the system comprising an anti-Cdld antibody pre-loaded into a hypodermic needle.
[00125] Clause 44. The system of clause 42 or 43, wherein the hypodermic needle is an autoinjector. EXAMPLES
[00126] The invention of present disclosure may be better understood by reference to the following examples, which should be understood as exemplary and non-limiting.
Example 1. Identification And Description Of Cdld-Dependent Neuroinflammation Impairment Of Tissue Repair Following Neurotraumatic Injury.
[00127] CD Id protein not only presents lipid antigens to natural killer T cells (NKT cells), but also exerts intrinsic signaling to regulate immune and inflammatory responses. Such CD Id activities have not been studied in the central nervous system (CNS), including the spinal cord. To investigate whether and which spinal cord cells express CD Id, immunofluorescence microscopy (IFM) analysis was performed of primary rat spinal cord cells and immunohistochemistry (IHC) analysis of rat spinal cord tissue sections. IFM analysis showed co-expression of CD Id and Ibal (a microglial/macrophage-specific marker), CD Id and Olig2 (oligodendrocyte transcription factor 2, a specific marker of oligodendrocyte progenitor cells or ODCs), or CDld and CD31 (the most sensitive marker for endothelial cells or ECs; Fig. 1, left panel), indicating that CDld is constitutively expressed on primary rat spinal cord microglia/macrophages, ODCs, and ECs. These results are in line with previous reports. CDld was not present on the surface of either neurons (i.e., NeuN+ cells) or astrocytes (i.e., GFAP+ cells) (Fig. 1, left panel). Our IHC analyses revealed the same distribution and phenotype of CDld expression (Fig. 1, right panel).
[00128] To investigate whether CDld protein expression was affected by a SCI, performed were IHC analysis of CDld in rat spinal cord tissues that were collected from rats on days 1, 3, and 7 post-SCI or rats with a sham injury (Fig. 2). Compared to sham-injured rats, CD 1 d gradually and markedly increased in the injured epicenter, both rostral and caudal, over the 7-day analysis following SCI (Figs. 3, 4). Thus, in the spinal cord, CDld is constitutively expressed on microglia/M^), ODCs, and ECs, but not on neurons or astrocytes. Thus, CDld expression is upregulated upon a SCI.
[00129] Materials and Methods.
[00130] Animals.
[00131] Adult C57BL/6J wildtype (WT) male and female mice and, Sprague Dawley (SD) adult and pregnant rats were purchased from Charles River, Inc. (Wilmington, MA). CDld knockout (CDldKO) mice on the C57BL/6J background were originally provided by Dr. Luc van Kaer (Vanderbilt University). Mice deficient in Type I NKT cells (Traj l8KO) on the C57BL/6J background were purchased from The Jackson Laboratory (Bar Harbor, ME); breeding was carried out at the Indiana University School of Medicine Laboratory Animal Resource Center. Male and female WT and CDldKO mice were used at 8 weeks of age. All mice were maintained on a 12- hour/12-hour light/dark cycle, with food and water ad libitum. For behavioral tests and histological analyses, 20 each of WT and CDldKO mice (half male and half female) were randomly divided into four groups: WT-sham, n=6; WT-SCI, n=14; CDldKO-sham, n=6 and CDldKO-SCI, n=14.
[00132] Primary spinal cord cell cultures.
[00133] The generation of primary spinal cord neurons followed methods which were published previously. X. Wu et al., RhoA/Rho Kinase Mediates Neuronal Death Through Regulating cPLA(2) Activation. Mol Neurobiol 54, 6885-6895 (2017). In brief, E15 rat spinal cords were isolated and dissociated in LI 5 medium (GE Healthcare Life Sciences, Cat. #41300039). The cells were cultured in Dulbecco’s Modified Eagle Medium (DMEM) supplemented with 10% fetal bovine serum (FBS), 5% horse serum and 2mM glutamine (GE Healthcare Life Sciences, Cat. #A1443101L) on 1.2 centimeters poly-L-lysine-coasted microscope coverslips in 5% CO2. Three days later, they were washed with 1 * phosphate-buffered saline (PBS) and fixed in very cold (-20°C) methanol for 10 minutes. After 3 washes with PBS, the cells were stored at 4°C and ready for immunofluorescence (IF) staining.
[00134] Glial cells were isolated from postnatal day 1 (Pl) rat spinal cords and cultured as previously described in the art. H. Lian, et al., Protocol for Primary Microglial Culture Preparation. Bio-Protocol 6(21) (2016) el989. First, the spinal cords were isolated from Pl rats, the cells were dissociated and cultured in DMEM containing 10% FBS and 2mM glutamine on coated T75 flasks. [00135] After 7 to 8 days, the astrocytes were confluent. The flasks were then shaken at 180 rpm for 1 hour on an orbital shaker to collect the microglia in the supernatant; these were then cultured in DMEM + 10% FBS and 2mM glutamine on coated microscope coverslips for 3 days. Twenty mL of fresh media were added to the flasks which were placed on an orbital shaker at 240 rpm for 6 hours for collecting the oligodendrocyte precursor cells (OPC) in the supernatant. The OPCs were cultured on coated coverslips for 3 days. The remaining astrocytes in the flasks were rinsed with PBS and incubated in 5mL of 0.5% trypsin (GE Healthcare Life Sciences; Cat. #25300054) for 5 minutes; 10 mL of fresh medium was then added. After collection and centrifugation, the astrocytes were placed on coated coverslips and cultured for 3 days. Finally, all of the microglia, oligodendrocytes and astrocytes were washed and fixed in cold methanol as described above. These fixed cells were stored at 4°C and ready for IF staining.
[00136] Contusive spinal cord injury.
[00137] A moderate contusive spinal cord injury (SCI) in mice was produced at the 10th thoracic vertebra (T10) by the Louisville Injury System Apparatus (LISA) impactor. Briefly, mice were anesthetized with ketamine (87.7 mg/kg) and xylazine (12.3 mg/kg) by an intraperitoneal (i.p.) injection. A midline skin incision was made from T8 to T12 over the spine.
[00138] The muscles were separated and the spine from the T8 to T12 vertebrae was exposed. Mice were placed in a U-shaped container and the T8 to T12 spinal area was fixed bilaterally with the LISA stabilizer. A T10 laminectomy was performed, and a SCI induced by the LISA impactor at a displacement of 0.40 cm, and a velocity 0.9 meters/millisecond was induced. Sham-injured control animals received the laminectomy without the SCI. The pressure of the nitrogen which controls the impact tip was set at 18 psi. After impact, the injured area was checked and the bleeding stopped. The muscles and skin were sutured with 3-0 silk surgical thread (Henry Schein, Melville, NY). Buprenorphine (0.01-0.05 mg/kg, in the slow-release form) and 1 mL saline were subcutaneously injected into the injured mice. The mice were then placed into a temperature- and humidity-controlled cage until recovery from the anesthesia. Wet food was provided during recovery. A manual bladder massage to void urine was performed twice daily until the reflex bladder function had returned.
[00139] In-vivo 5-bromo-2'-deoxyuridine (BrdU) labeling.
[00140] Thymidine analog 5-bromo-2'-deoxyuridine (BrdU) may be used as a label to measure cell proliferation. WT and CDldKO male and female mice (8 weeks old; 4 mice: half male/half female mice per group) were given BrdU in their drinking water (5g/L) and regular food immediately after the T10 contusive SCI for one week as previously described. The animals were then sacrificed, and the spinal cords were collected. Cryostat longitudinal sections (25 pM) were then cut for the histological assessments described in the text. [00141] Cytokine assay.
[00142] Six groups of mice: WT naive, sham, SCI and CDldKO naive, sham and SCI on days 1, 3, 7, and 35 post-SCI were used. At least 4 mice (half male, half female) per group were included at each time point. Each mouse was anesthetized as above. A perfusion needle was inserted into the left ventricle and the right atrium was cut. Thirty mL of 0.0 IM phosphate-buffered saline (PBS, pH7.4) was pumped continuously into the left ventricle, coursing throughout the whole animal, until which time only PBS was observed to flow from the right atrium. Then, a 1- centimeter piece of spinal cord containing the injured epicenter was removed and placed individually into a tube which contained 200 pL of PBS (0.01M). The spinal cords were homogenized thoroughly and placed on ice for 30 minutes. The tubes were then centrifuged at 40,000 rpm at 4°C for 15 minutes. The supernatants were collected for the detection of cytokines via a multiplex magnetic bead array.
[00143] Cytokines and chemokines (32 in total) in mouse spinal cord homogenates were measured using the Milliplex® MAP Mouse Cytokine/Chemokine premixed 32 magnetic bead panel (Millipore) following the manufacturer’s instructions. The data were acquired on a Luminex platform by the Indiana University School of Medicine’s Simon Cancer Center Multiplex Analysis Core and were analyzed by MILLIPLEX® Analyst 5.1 software (Millipore).
[00144] qPCR of spinal cord mRNA.
[00145] Total RNA was isolated using an RNAeasy kit (Qiagen) according to the manufacturer’s protocol and quantified by UV spectroscopy. Random hexamers and reverse transcriptase were used for cDNA synthesis (Roche Applied Science Transcriptor High Fidelity cDNA Synthesis Kit). Predesigned TaqMan probes for murine CDld (Mm00783541) and GAPDH (Mm99999915_gl) were purchased from Applied Biosystems. CDld and GAPDH transcripts were quantified using a TaqMan PCR Master Mix and ABI Prism 7000 instrument (Applied Biosystems). The PCR profile used was 95°C for 20 seconds (1 cycle; hold stage), 95°C for 1 second and 60°C for 20 seconds (40 cycles; PCR stage). The relative change in cdldl gene expression between the sham and SCI groups was calculated using the ACt method (7) and represented as the fold change, which was normalized to the sham group.
[00146] Behavioral assessments. [00147] Four behavioral assessments, the Basso Mouse Scale (BMS) (D. M. Basso et al., Basso Mouse Scale for locomotion detects differences in recovery after spinal cord injury in five common mouse strains. J Neurotrauma 23, 635-659 (2006)), Grid-Walking (O. Y. Chao, et al., The grid-walking test: assessment of sensorimotor deficits after moderate or severe dopamine depletion by 6-hydroxydopamine lesions in the dorsal striatum and medial forebrain bundle. Neurosci 202, 318-325 (2012)), Rotarod (R. J. Hamm, et al., The rotarod test: an evaluation of its effectiveness in assessing motor deficits following traumatic brain injury. J Neurotrauma 11, 187— 196 (1994)) and transcranial magnetic-motor evoked potential (tcMMEP) (R. D. Linden et al., Magnetic motor evoked potential monitoring in the rat. J Neurosurg 91, 205-210 (1999)), were used to evaluate hind limb functional recovery. Prior to surgery, each mouse was trained with the open field (BMS), Grid-Walking, and Rotarod every day for 3 days. The baselines of the behavioral tests were recorded.
[00148] Basso Mouse Scale (BMS).
[00149] The BMS locomotor test was performed before the SCI and on days 1, 3, 7, 14 and 28 post-SCI, via a method published previously (Basso et al. (2006)). Each mouse was placed in a 42-inch diameter open field and observed for 4 minutes by two evaluators who were blinded as to the experimental groups. The movements were scored based on hind limb joint movement, weight support, plantar stepping, paw position, coordination of all four limbs, and trunk and tail control following the standard guide. The BMS scores were classified on a scale of 0 to 9 (0=complete paralysis; 9=normal locomotion).
[00150] Grid-Walking.
[00151] The Grid-Walking test assesses the sensory-motor coordination ability of the mouse
(Chao et al. (2012)). Briefly, an elevated metal rectangular grid (12 x 36 in2, with each grid a square of 0.5 x 0.5 in2) was placed 16 inches above a table. Each mouse was placed in the center of the grid for 3 minutes and their walking was recorded. The total number of foot-steps and foot drop errors of each hind limb were counted by observers who were blinded as to the experimental groups. A foot drop error was counted when the hind limb paw completely dropped through the grid with all toes and the heel below the grid surface. The percentage of foot drop errors was then calculated. This test was performed on the day before surgery and 28 days post-SCI. Only those mice showing consistent plantar stepping (BMS > 5) were tested on the grid.
[00152] Rotcirod.
[00153] The Rotarod tests balance, grip strength and motor coordination (Hamm et al. (1994)). Each mouse was placed on a rotating rod and the latencies on the rod (i.e., time until the mice come off the rod) were recorded at two different speeds (18 rpm and 30 rpm), both before injury and 28 days after injury.
[00154] Transcranial magnetic motor-evoked potentials (tcMMEP).
[00155] The conductivity of the descending axonal pathways from the brain to the spinal cord was assessed by the tcMMEP method, an in vivo electrophysiological measure of motor pathway integrity which has been published previously (Linden et al. (1999)). Briefly, before the SCI and 28 days post-injury, an electromagnetic coil was placed over the cranium and a muscle twitch sensor connected to the gastrocnemius. The current was turned on; the action potentials stimulated subcortical structures which descended down to the ventral spinal cord/synapse and motor-neuron pools. The output signals were detected and recorded from the gastrocnemius muscles of both hind limbs.
[00156] Histological Assessments.
[00157] Spinal cord sample collection and cryostat section generation. Mice were anesthetized and perfused with 30 mL of 1*PBS (0.01M) and 30 mL of 4% paraformaldehyde (PFA). A 3-cetimeter segment of the spinal cords which included the injury epicenter was dissected, removed and placed in 4% PFA overnight. The next day, the spinal cord segments were transferred into a 30% sucrose solution in 1 *PBS for one week. One centimeter of spinal cord around the injury epicenter was embedded with optimal cutting temperature medium (“OCT embedding medium”, or “OCT medium”) (Fisher Healthcare, Waltham, MA) and cut into 25 pm thick cross or longitudinal sections, which were mounted on frosted slides in five identical sets, eight slides per set, 7-8 sections per slide.
[00158] Lesion area and spared white matter measurement. One set of sections/ slides was stained with Cresyl Violet Eosin for measuring the lesion area, lesion volume and viable neurons; another set was stained with Luxol Fast Blue (LFB) for the assessment of the spared white matter area and volume. For lesion area quantification, the epicenter of each spinal cord was selected, the cord area and lesion area were outlined, and measured with an Olympus BX60 microscope (Olympus, Tokyo, Japan) using Neurolucida Neuron Tracing Software (MicroBrightField, Williston, VT). The lesion area (displayed as a percentage) was calculated as the lesion area divided by the entire spinal cord area x 100. For spared white matter (SWM), the epicenters of the injured spinal cords were selected and the area of SWM, which stained blue, was also measured using the Neurolucida software. The SCI SWM areas were compared to WT and CDldKO sham white matter areas; the SWM areas (presented as percent) were then calculated.
[00159] 3-Dimensional lesion and SWM volume assessments.
[00160] The epicenters of the lesions were set as a middle (zero) point and 0.3-millimeter intervals, from the rostral to caudal areas of the spinal cord. There were, in total, 11 lesion areas/SWM collection sites which are presented as follows (in millimeters): rostral 1.5, 1.2, 0.9, 0.6, 0.3, 0, 0.3, 0.6, 0.9, 1.2, 1.5 caudal. The lesion areas/SWM of each section which contained the lesion area at these locations were measured as: Al, A2, A3, etc.
[00161] The lesion and SWM volumes were calculated as: total volume = (A1+A2+A3+.. .) x lesion length, following published methods. See R. P. Michel & L. M. Cruz-Orive, Application of the Cavalieri principle and vertical sections method to lung: estimation of volume and pleural surface area. J Microsc 150, 117-136 (1988). The SCI length was calculated from the location at which the first section showed a lesion, to the location that was the end of the lesion from the rostral to caudal sides. A 3 -Dimensional model was reconstructed with the Neurolucida® System based on the lesion areas of each location, following the instructions of the Neurolucida® System Software.
[00162] Immunofluorescence.
[00163] A standard immunofluorescence staining protocol was used. Briefly, the coverslips of cells or slides with tissue sections were incubated in 0.3% Triton X-PBS (PBST) twice for 15 minutes each, then incubated with 10% goat serum (Cell Signaling Technology, Cat. #5425) in PBST for 1 hour. The first antibodies were applied on the coverslips and slides overnight at 4°C. The primary antibodies used were specific for CDld (1 :500; Biolegend, 205802), pill-tubulin (1 : 1000; Millipore, AB9354), NeuN (1 :800; Millipore, ABN78), GFAP (1 : 1000; Abeam, ab4674), Iba-1 (1 :500; Abeam, abl78846), CD31 (1 :500; ThermoFisher, PA5-16301) and Olig2 (1 :500; Millipore, AB9610). The next day, the coverslips and slides were washed 3 times with PBST (10 min/wash) and incubated with the secondary antibodies, Cy3 goat anti-rat IgG and Alexa Fluor488 anti-rabbit (Jackson Immunolabs, 112-166-143 and 111-545-003, respectively) for 1 hour at room temperature. After an additional 3 washes with PBST, 5pg/ml Hoechst (Cell Signaling Technology, #4082) in PBS was applied for 5 minutes and washed twice with PBS (5 min/wash). Fluoromount-G (SouthernBiotech, 0100-01) was used as the mounting medium. For double staining with BrdU, the tissue sections were first stained with Iba-1 and secondary antibodies, and then BrdU staining as follows: the sections were rinsed three times with 1*PBS (10 minutes each) and then briefly washed with ddH2O. The slides were then incubated in 2N HC1 for 60 minutes, washed with ddH2O, and incubated in a borate buffer solution (0.1M, pH8.4) for 10 minutes. The slides were then washed three times in PBS (30 min/wash) and blocked with blocking buffer (5% normal goat serum/1% bovine serum albumin/0.1% Triton X100 in PBS) overnight at 4°C. The next day, an anti-BrdU antibody (1 :600; Abeam, ab6326) was diluted in blocking buffer and the slides were incubated overnight at 4°C. The following day, after 3 washes, the secondary antibody (Cy3 goat anti-rat IgG) was applied and the slides were incubated overnight at 4°C. Finally, the slides were washed with PBS, incubated with Hoechst and mounted with fluoromout-G. After staining, images of the cells and sections were taken with an Olympus BX60 microscope, an Olympus BX61 confocal microscope (FLUOVIEW™, FV1200, Japan) and analyzed with ImageJ software (NIH).
[00164] In-vivo treatment with CD Id-specific antibody.
[00165] Adult C57BL/6J WT male and female mice (8 weeks old; 18 mice each) were randomly divided into two groups: SCI that received an isotype control antibody (TW2.3) (H. Yuwen, et al., Nuclear localization of a double-stranded RNA-binding protein encoded by the vaccinia virus E3L gene. Virology 195, 732-744 (1993)), mouse IgG2b, and those receiving the anti-mouse CDld-specific mAb, 1H6, generated previously (T. J. Roberts et al., Recycling CDldl molecules present endogenous antigens processed in an endocytic compartment to NKT cells. J Immunol 168, 5409-5414 (2002).). All of the mice received BMS, Grid-Walking and Rotarod training before a T10 contusive SCI. The mAbs were injected intraperotineally one day before injury and 10 minutes after, and 3, 7, 14, 21, 28 and 35-days post-SCI. The BMS was performed on days 1, 3, 7, 14, 21, 28, 35, and 42 after SCI. The Grid-Walking and Rotarod tests were evaluated on day 42 or 43 post-SCI. All mice were sacrificed on day 45 following SCI and spinal cord samples were collected as described above. Serial cryostat cross sections were cut and collected on the slides, and performed as above.
[00166] Preparation of single cell populations from mouse spinal cord and liver.
[00167] Sham-, and spinal cord-injured WT mice (days 1, 3 and 7 post-SCI) were anesthetized and perfused with 0.01M PBS as above. For each mouse, a 2-centimeter section of spinal cord which contained the injured site was collected and used to generate a single cell suspension. Briefly, the spinal cord was cut and minced into small pieces in Hanks’ Balanced Salt Solution (HBSS) with calcium and magnesium, and fdtered through 70 pm cell strainers. After being pelleted at 700xg for 10 minutes, the cells were resuspended in 10 m of 40% Percoll (GE Healthcare, Chicago, IL) and centrifuged again at 950*g for 20 minutes at room temperature with the brake off. The cell pellets were then used for flow cytometric analysis of the immune cells present in the spinal cord.
[00168] To prepare liver mononuclear cells, after perfusion, the liver was collected and ground through 70 pm cell strainers using the end of a 5 mb syringe. The cells were centrifuged at 700*g for 10 minutes and then resuspended in lOmL of 40% Percoll, where they were centrifuged again at 950*g for 20 minutes at room temperature with the brake off. The cell pellets were resuspended in 5 mb of red blood cell (RBC) lysis buffer (BioLegend, San Diego, CA) for 5 minutes at room temperature to lyse the RBCs. After centrifugation at 700*g for 10 minutes at 4°C, the liver mononuclear cells were used for flow cytometric analysis.
[00169] Flow cytometry.
[00170] Single-cell suspensions of spinal cord and liver were stained to determine the frequency of immune cells in these tissues. Briefly, cells were incubated with an FcRy-blocking CD16/CD32-specific antibody (eBioscience, San Diego, CA) for 15 minutes, followed by staining with fluorochrome-conjugated antibodies against mouse CD45, CD1 lb, Ly-6G, CD45R, CD3, and NK1.1. All antibodies were purchased from BioLegend (San Diego, CA). The cells were then stained with the Fixable Viability Dye eFluor™ 780 (FVD, eBioscience) to exclude FVD-positive dead cells. After washing, cells were fixed in 2% PFA and subjected to flow cytometry. The data were analyzed using FlowJo vlO software (Tree Star, San Carlos, CA). Spinal cord leukocytes were defined as CD45Hlgh cells that could be distinguished from CD45Low microglia. Within the CD45Hlgh population, Ly-6G expressing cells were defined as polymorphonuclear neutrophils (PMNs). Among the non-PMN leukocytes, lymphocytes were identified as CDl lb'CD45Hlgh and M/M(|) as CD45HlghCDl lb+. Lymphocytes, excluding the CD45R+ B cells, were subdivided into NK, NKT, and non-NKT cells based on the expression of NK1.1 and CD3.
[00171] a-Galactosylceramide (a-GalCer) treatment.
[00172] Twenty-eight adult (8 weeks) C57BL/6J WT mice (14 male, 14 female) were randomly assigned into 4 groups: sham+PBS, N=6; sham+ a-GalCer, N=6; SCI+PBS, N=8 and SCI+ a-GalCer, N=8. Before the SCI, all mice received BMS, Grid-Walking and Rotarod training 3 times. They then received a T10 moderate contusive SCI with the LISA impactor. Five minutes after the SCI, every day the first week and twice a week from the 2nd week to 6th week, 100 pl (200pg/mouse) of a-GalCer (ENZO Life Sciences, Farmingdale, NY) was injected intraperotineally into the appropriate groups of mice. As the vehicle control, the appropriate mice received 100 pL of 1 *PBS, intraperotineally. The BMS tests were performed on days 1, 3, 7, 14, 21, 28, 35 and 42 post-SCI. The Grid-Walking and Rotarod tests were assessed 6 weeks after the SCI.
[00173] Trajl8KO mouse SCI study.
[00174] Eight C57BL/6 background Traj 18KO (Type I NKT cell-deficient) mice and eight wildtype (WT) littermates received training and surgery as above. The baseline behavioral scores and tcMMEP electrophysiology were recorded. The BMS was tested on days 1, 3, 7, 14, 21, 28 and 35 post-SCI. Grid-Walking, Rotarod and tcMMEP were assessed 5 weeks after injury.
[00175] Statistics.
[00176] The statistical parameters, including the definitions and exact values of the number of animals, standard deviations, /^-values, and the types of the statistical tests that were performed are reported in the corresponding figure legends. Statistical analyses were carried out using GraphPad Prism software (version 7.00, La Jolla, CA) or R Studio. Comparisons between the experimental groups were performed by a one-way or two-way ANOVA with Tukey’s post-hoc test, or Student’ s t-test as indicated. A /2-value below 0.05 was considered significant.
Discussion.
[00177] Example 1 herein provides compelling evidence that CD Id, an MHC class I-like molecule mostly known for presenting lipid antigens to NKT cells, plays an important role in the neuropathology that occurs following a neurotrauma, particularly an SCI. It was found that CD Id was constitutively expressed in the spinal cord on microglia/M<^, ODCs, and ECs, but not on either neurons or astrocytes. Moreover, this CDld expression was upregulated after a SCI. In the spinal cord post-injury, as compared to WT, CDldKO mice exhibited less tissue damage, reduced activation and proliferation of microglia/M^), and lower levels of proinflammatory cytokines, but higher levels of anti-inflammatory cytokines and increased frequencies of tissue-repairing M$. Consequently, CDldKO mice had substantially improved post-SCI functional recovery versus WT mice.
[00178] The contribution of CDld in spinal cord neuropathology after a SCI occurs in a manner independent of the conventional CDld/NKT cell axis because: (1) the frequency of NKT cells was extremely low or negligible (~0.17%) in spinal cord tissues of WT mice with or without a SCI, (2) spinal cord-injured WT mice receiving a-GalCer, a glycolipid that when presented by CDld strongly activates NKT cells (Sriram et al. (2005)), were no different in their motor function and behavioral assessment scores when compared to the control group, suggesting in-vivo activation of NKT cells by a-GalCer had no significant impact on the functional and behavioral deficits observed in WT mice post-SCI, and (3) there were no differences in motor function and behavioral assessments after a SCI between WT mice and C57BL/6 Traj 18KO mice, with the latter selectively lacking Type I NKT cells. These results suggest that the CD Id-associated neuropathology following SCI is mediated by CDld intrinsic signaling or heretofore-unknown mechanisms rather than the canonical CDld/iNKT cell axis.
[00179] As an MHC class I-like glycoprotein that is primarily expressed on antigen- presenting cells (APCs), CDld consists of two polypeptide chains (a and |32-microglobulin or 02M) (see Fig. 41). CDld interacts with T cell receptor (TCR) on the surface of NKT cells to present lipid antigens. In contrast to the classical MHC class I molecules that are highly polymorphic, CDld molecules exhibit limited sequence diversity. CDld has a short cytoplasmic tail containing a putative tyrosine-dependent signal motif YXXZ (where Y signifies tyrosine, X is any amino acid, and Z is a hydrophobic amino acid). Crosslinking human CDld on the surface of myeloid cells induces tyrosine phosphorylation and cytokine production. These crosslinking effects are abolished when the CDld cytoplasmic tail is replaced with a CD la cytoplasmic domain, suggesting that CDld exerts intrinsic signaling via its YXXZ motif in the cytoplasmic tail. Another study also revealed that CDld intrinsic signaling in macrophages controls NLRP3 inflammasome expression during inflammation in an animal model of dextran sodium sulfate (DSS)-induced colitis. (S. Cui et al., CDldl intrinsic signaling in macrophages controls NLRP3 inflammasome expression during inflammation. Sci Adv 6(43) (2020) eaaz7290.) Mechanistically, CDld engagement with the natural glycosphingolipid ligand iGb3 induces intracellular Ser-330 dephosphorylation, which reduces AKT-dependent STAT1 phosphorylation and subsequent NF- KB activation, eventually leading to the transcriptional down-regulation of NLRP3 inflammasome expression in macrophages in dextran sodium sulfate (DSS)-induced colitis. Therefore, CDld not only binds and presents lipid antigens for recognition by NKT cells but also exerts intrinsic signaling to regulate inflammatory and immune responses. Given the findings described herein that CD Id-associated neuropathology following a SCI was independent of the canonical CDld/iNKT cell axis, CDld intrinsic signaling was likely triggered. In this regard, CDldKO and WT mice post-SCI could be used to investigate the pathogenic role of CDld intrinsic signaling in SCI-triggered sterile neuroimmune and neuroinflammatory responses in the spinal cord, which warrants further studies.
[00180] In mice, there are two broad subsets of M/Mc[) known as inflammatory (CD45+CD1 lb+Ly-6G-Ly-6CHigh) and tissue-repairing (CD45+CD1 lb+Ly-6G-Ly- 6CLowF4/80High). (See, e.g., R. M. Kratofil, et al., Monocyte Conversion During Inflammation and Injury. Arterioscler Thromb Vase Biol 37(1), 35-42 (2017).) During inflammation or tissue injury, M/M<j) responses are characterized by the accumulation of inflammatory M/M([) in the first phase to clear cellular debris, followed by the conversion to tissue-repairing M/M^> in the reparative phase that contributes to tissue repair and wound healing. The phenotypic switch from inflammatory M/M<^ to tissue-repairing M/M^ is mainly driven by IL-4 at the site of inflammation or injury, which is more efficient than systemic differentiation factors.
[00181] Inflammatory M/Mc[) express higher levels of proinflammatory cytokines such as IL-1, IL-6, IL-8, and TNF-a during the early phase of inflammation or tissue injury, whereas tissue-repairing M/M<j) produce growth factors such as VEGF and anti-inflammatory mediators (e g., IL-10 and TGF-pi) that foster inflammation resolution, tissue repair and wound healing. Strikingly, compared to WT mice, it was found, as described herein, that CDldKO mice had a significantly higher frequency of tissue-repairing M/M([) in injured spinal cord tissues in the postacute phase of SCI. Indeed, the frequencies of inflammatory and tissue-repairing M/M([) displayed opposite temporal patterns in injured spinal cord tissues from both WT and CDldl KO mice over the 28-day course of SCI described herein. Specifically, the frequencies of inflammatory M/M<[) were high in the initial phase of SCI and then gradually declined, whereas the frequencies of tissuerepairing M/M were low in the early phase of SCI and then increased over time. However, day 7 post-SCI was a turning point where the frequency of tissue-repairing M/M<[) was significantly higher in injured spinal cord tissues from CDldKO mice than those from WT. In contrast, the frequencies of both inflammatory and tissue-repairing M/M([) in WT and CDldKO mouse livers remained unchanged over the 28-days post-SCI. These results suggest that M/M$ undergo differentiation and functional changes in response to local tissue-specific factors rather than from systemic signals. Concomitantly, day 7 post-SCI was also a turning point in CDldKO versus WT mice when inflammation and lesions in spinal cord tissue were significantly reduced, whereas motor function was markedly improved. Thus, tissue-repairing M/M$ appear to be derived by local factors in the spinal cord microenvironment and make a substantial contribution to tissue repair and wound healing of the injured spinal cord in CDldKO mice following a SCI.
[00182] It was also found that CDldKO mice had higher levels of IL-4, IL-7, and VEGF in injured spinal cord tissues post-SCI than WT mice. IL-4 is a well-known anti-inflammatory cytokine and also orchestrates the differentiation of inflammatory M/M<^ into tissue-repairing
Interleukin-7 (IL-7) promotes both neuronal survival and neuronal progenitor cell differentiation and VEGF is one of the most important proangiogenic cytokines, promoting cellular reactions throughout all phases of the wound healing process. Thus, the elevated levels of IL-4, IL-7, and VEGF in CDldKO injured spinal cord tissues induce the polarization of inflammatory M/M<[) into tissue-repairing M/M([) and the consequent tissue-repairing benefits. In addition to the tissue milieu in which IL-4 amplifies tissue-repairing M/M^ polarization, Nr4al (nuclear receptor subfamily 4 group A member 1, also known as Nur77) is a transcription factor that not only plays a key role in the differentiation of tissue-repairing M/M<[) from inflammatory
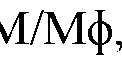
but it is also essential for the survival of tissue-repairing M/M<^. The scRNA-seq analysis described herein (Fig. 39) revealed that Nr4al was highly expressed in M<j), especially in those from WT or CDldKO mice following a SCI. In addition, VEGF-A was predominantly produced by M2 rather than Ml Mij). Thus, abrogation of CDld signals likely upregulates SCI-induced gene expression of VEGF- A and Nr4al, thereby increasing survival and differentiation of M2 macrophages (or tissuerepairing M/Mcj)) to promote tissue repair and regeneration. Of note, the levels of MCP-1 (also known as CCL2) in injured spinal cord tissues from WT and CDldKO mice post-SCI were comparable (see Table 1, below).
[00183] Given that MCP-1 is a principal chemokine for recruiting Ly-6CHlgh M/M(|) into inflammatory sites through its binding to its receptor CCR2 that is highly expressed on inflammatory Ly-6CHlgh M/M(|), the similar levels of MCP-1 in injured spinal cord tissues from CDldKO and WT mice explain the comparable frequencies of inflammatory M/M(|) found in the acute phase of SCI in these groups.
[00184] CD Id ligation alone, in the absence of NKT cells, can rapidly trigger activation of the NF-KB pathway in CDld+ cells such as human M/M(|) and dendritic cells (DCs), and subsequently induces cytokine production and inflammasome activation. These findings imply that surface CD Id on M/M(|), oligodendrocytes (ODCs), and endothelial cells (ECs) in the spinal cord can be directly engaged by lipids released from damaged tissues and cells post-SCI. In this regard, direct interaction of CD Id with lipids can be blocked. Indeed, it was found that administration of anti-mouse CDld blocking mAb via i.p. injection to WT mice before and after SCI ameliorated CD Id-mediated spinal cord neuropathology. Treatment with this anti-CDld mAb significantly increased BMS scores, decreased the hind limb foot drop error rate and increased latency on the Rotorod 6 weeks post-SCI. IHC analysis also showed that treatment with the antimouse CDld mAb significantly reduced the size of the lesion area and protected white matter. In fact, treatment with anti-mouse CDld mAb had comparable neuroprotective effects as those observed in CDldKO mice, thereby representing a potential immunotherapeutic strategy for SCI. [00185] Taken together, the results described herein suggest that a neurotrauma such as SCI triggers a Type I NKT cell-independent, CD Id-dependent neuroimmune/neuroinflammatory response that recruits inflammatory M/M<[) into inflamed spinal cord tissues during the early phase of SCI. In a CD Id-deficient environment, there would be reduced CD Id-intrinsic proinflammatory signaling, but an increase in IL-4 production that promotes the differentiation of inflammatory M/M<[) into the tissue-repairing M/M<[). Tissue-repairing M/M$ produce high levels of IL-4 and VEGF which counteracts neuroinflammation and improves tissue repairing, respectively. Therefore, these findings provide fundamental insight into the mechanisms underlying neurotrauma-triggered sterile neuroimmune/neuro-inflammatory responses in the spinal cord. Targeting CDld may represent a novel therapeutic strategy to reduce neurological damage and improve functional recovery after a SCI.
Experiment 1. CDldKO mice exhibit less spinal cord damage after a spinal cord injury.
[00186] Similar to the rats, increased CDld expression over 7 days was detected in mouse spinal cord tissues following a T10 moderate contusive SCI (Fig. 5). To analyze the effects of CDld on histological outcomes of the spinal cords from mice post-SCI, sections were stained with Cresyl Violet (also known as Nissl) and Luxol Fast Blue (LFB) to evaluate lesion area size and amount of spared white matter (SWM) (Fig. 7), respectively. On day 7 post-SCI, in WT mice, the averages of lesion size and SWM volume were 41.1% and 35.2%, respectively (Fig. 6). In CDldKO mice, they were 23.8% and 53.3%, respectively (Fig. 6). Thus, spinal cords of CDldKO mice had less damage and greater amounts of SWM than WT mice after injury. 3-Dimensional reconstruction were generated of the injured spinal cord areas based on Nissl and LFB staining data (Figs. 8A-8B). As is evident, compared to WT mice, injured spinal cords from CDldKO mice had a smaller lesion volume and a shorter length of spinal cord damage, and a greater volume of SWM after SCI (Figs. 8A-8B, 10A-10C). Thus, CDldKO mice exhibit less spinal cord tissue damage than WT mice after a SCI.
[00187] With less spinal cord damage in CDldKO mice post-SCI, it was speculated that viable motor neurons might show enhanced protection. IHC analysis was performed of viable motor neurons in the spinal cords of CDldKO versus WT mice post-SCI. There were almost no viable motor neurons in the ventral horns at the injury epicenters of both WT and CDldKO mice post-SCI (Figs. 9, 11). However, in marked contrast, 1.0 mm both rostral and caudal from the injured epicenter, CDldKO mice had a significantly greater number of viable motor neurons than WT mice post-SCI (Figs. 9, 11). Thus, a CDld deficiency confers protection to spinal cord neurons surrounding the SCI impact core. These results suggest that CDldKO mice can be used as an animal model to study secondary injury progression in the traumatic penumbra containing viable tissue around the irreversibly damaged SCI core. Additionally, the results highlight rationales to study penumbral pathophysiology, which may lead to the development of novel therapeutic strategies to salvage penumbral spinal cord tissue following a neurotrauma such as an SCI. Experiment 2. A CDld deficiency enhances locomotor functional recovery following a spinal cord injury.
[00188] Because it was found that CDldKO mouse spinal cords had less tissue damage and neuronal cell death after a SCI, it was investigated whether they also had a better functional recovery than WT mice post-injury. Thus, both WT and CDldKO mice were subjected to a T10 moderate contusive SCI as described above. After the SCI, the mice were subjected to the Basso Mouse Scale (BMS), Grid-Walking, and Rotarod tests for evaluating locomotion, sensorimotor, and motor-coordination functions (Figs. 12, 13), respectively. On days 1 and 3 post-SCI, both WT and CDldKO mice had similarly low BMS scores with complete paralysis of their hind limbs (Fig. 14). However, on day 7 post-SCI, CDldKO mice started to show significantly improved hind limb functional recovery compared to WT mice (Fig. 14). This striking difference was also evident in videos showing the relative mobility of WT and CDldKO mice 3 weeks post-SCI. This broad and important difference in the level of functional recovery improvement continued for over 4 weeks of BMS examination. Thus, CDldKO mice exhibit a better locomotor functional recovery from a SCI than do WT mice.
[00189] The Grid-Walking test showed that both WT and CDldKO mice post-SCI had an increased number of foot drop errors than those with a sham injury (Fig. 15 A). Compared to WT mice with a foot drop error rate of 26.7%, CDldKO mice had a significantly lower foot drop error rate (15.0%) (Fig. 15A), which constituted a 44% improvement over WT mice (p<0.01). Therefore, CDldKO mice have a much greater recovery of sensory-motor coordination than WT mice after a SCI.
[00190] The Rotarod test showed that both WT and CDldKO mice had a lower latency rate post-SCI than sham-injured mice over the 28-days of analysis (Fig. 15B). When compared to WT, CD 1 dKO mice had a significantly longer latency (Fig. 15B), suggesting CD 1 dKO mice have better motor coordination recovery post-SCI.
[00191] Transcranial magnetic motor-evoked potentials (tcMMEP) were used to assess the functional integrity of the spinal cord’s descending axonal pathways in both WT and CDldKO mice following a SCI (Fig. 16). WT mice exhibited a prolonged latency of the evoked potential (Fig. 17A), a lower total response rate (Fig. 17B), and a reduced potential amplitude (Fig. 17C) post-SCI as compared to sham-injured WT mice. In marked contrast, injured CDldKO mice only exhibited a reduced potential amplitude but did not show a difference in either latency or response rate than sham-injured (Figs. 17A-17C, 18A-18D). Thus, of the three indicators of tcMMEP, spinal cord-injured CDldKO mice only have a reduced potential amplitude, whereas WT mice exhibit deficits in all three post-SCI. This suggests that a CD Id-deficient environment in vivo preserves the descending axonal electrophysiological pathways in mice after a SCI.
[00192] Taken together, compared to WT, CDldKO mice exhibit a remarkable improvement in locomotor functional recovery, sensory-motor coordination and balance, grip strength, hind limb motor coordination and functional integrity of the spinal cord post-injury, indicating that CD Id negatively affects the functional outcomes of a SCI.
Experiment 3. Microglia/M<|> of CDldKO mice are less activated and have reduced proliferation in the injured spinal cord.
[00193] Following a traumatic SCI, microglia/M$ undergo activation, proliferation and changes in gene expression and morphology, exerting either detrimental or beneficial effects. Given that spinal cord microglia/M<^ constitutively expressed high CD Id levels and this expression was further upregulated by an SCI, it was hypothesized that CD Id had an impact on microglial/M<[) responses to a SCI. To test this hypothesis, IHC was performed to analyze and compare the activation and proliferation of microglia/M$ by quantifying Ibal expression and in-vivo incorporation of BrdU in injured spinal cord tissues collected from WT and CDldKO mice on day 7 post-SCI. In both WT and CDldKO mice, Ibal expression intensity and Ibal+ cell numbers were dramatically greater than those in sham-injured mice (Figs. 19, 20A-20C), indicating that a SCI triggers activation and proliferation of microglia/M^). Interestingly, injured CDldKO mice had fewer numbers of Ibal+ microglia/macrophages in the injured epicenter and 0.5 - 1.0 mm rostral and caudal from the injury site as compared to WT mice (Figs. 19, 20A-20C). This suggests that CD Id signaling promotes microglial/M$ proliferation. Similarly, as compared to injured WT mice, CDldKO mice post-SCI had lower numbers of BrdU+ or BrdU+Ibal+ microglia/macrophages in the injured epicenter as well as 1.0 mm both rostral and caudal from the injury site (Figs. 19, 20B-20C). To confirm these results, scRNA-seq was used to analyze and compare transcriptomes of spinal cord cells from WT and CDldKO mice with or without a SCI (Figs. 38-40). Microglia were highly activated in the spinal cords of WT mice following a SCI compared to sham-injured WT mice. In WT mice, injured CDldKO mice expressed much lower levels of genes involved in microglia activation (Fig. 39), confirming that CDld is associated with microglia activation as revealed in our IHC analysis. Thus, CD Id-dependent signaling likely promotes the activation and proliferation of microglia/M^) in the spinal cord post-SCI. The lack of CD Id would then reduce activation and proliferation of microglia/M$, which may be responsible for the neuroprotection that was observed in injured CDldKO mice.
Experiment 4. A CDld deficiency promotes the polarization of macrophages into those involved in tissue repair and the anti-inflammatory response in the spinal cord post-SCI.
[00194] A SCI triggers sterile neuroimmune/neuroinflammatory responses dominated by tissue-resident microglia and M/M<[). These neuroimmune/neuroinflammatory responses can have both beneficial and detrimental effects. Here, M/M([ were analyzed and compared, as well as other types of immune cells in WT and CDldKO spinal cords following a SCI. CDldKO and WT mice post-SCI had a comparable percentage of neutrophils, B cells, total T cells, CD4 T cells, and CD8 T cells in the injured spinal cord (Figs. 36, 37). Notably, the frequency of NKT cells (CD451 CD3 'NK1.11 T cells) was extremely low or negligible in spinal cord tissues (<0.17%) from WT mice with or without a SCI, but high (-35%) in their livers (Fig. 37), suggesting that NKT cells act as key participants in liver physiology and pathology, but not in the spinal cord. In sharp contrast, the frequencies of spinal cord M/M^, including inflammatory (CD45+CD1 lb+Ly-6G'Ly- 6cHigh)ancj tissue-repairing (CD45+CD1 lb y-6G-Ly-6CLowF4/80Hlgh), were dramatically affected by a SCI in both CDldKO and WT mice. The frequencies of inflammatory and tissuerepairing M/M<[) displayed opposite temporal patterns in injured spinal cord tissues from both WT and CDldl KO mice over the 28-day analyses post-SCI (Figs. 21, 22A-22B); specifically, the frequencies of inflammatory M/Mc|) were high in the initial acute phase of SCI and then gradually declined, whereas the frequencies of tissue-repairing M/M<^ were low in the early phase of SCI and then increased over time (Fig. 22A). Interestingly, day 7 post-SCI is a pivotal turning point where the frequencies (%) of tissue-repairing M/Mc|) are significantly higher in injured spinal cord tissues from CDldl KO vs. WT mice (Fig. 23). In contrast, the frequencies of both inflammatory and tissue-repairing M/M<[) in livers from WT and CDldKO mice remained unchanged over the 28-day period post-SCI (Figs. 21, 22B). Therefore, these results suggest that M/M<|) undergo differentiation and functional changes in response to the local spinal cord tissue-specific environment rather than systemic factors. Concurrently, day 7 post-SCI (post-acute phase) was also a turning point, when spinal cord lesions were significantly reduced while motor functions were markedly improved in CDldlKO as compared to WT mice (Figs. 14, 15A-15B, 17A-17C, 18A-18D). Thus, in CDldKO mice, tissue-repairing M/M$ appear to be locally-derived and substantially contribute to tissue repair and wound healing of the injured spinal cord following a SCI.
[00195] To better understand the local tissue microenvironment that might determine the effects of CD Id on neuroimmune responses and the polarization of tissue-repairing M/Mj) in response to a SCI, spinal cord tissue levels of 32 cytokines, chemokines and growth factors were simultaneously quantified using a multiplex immunoassay kit. After an extensive perfusion of the mice with PBS, we harvested a 1 -centimeter section of spinal cord (including the SCI site) for extracting proteins that were used for the multiplex immunoassay. Compared to naive or sham conditions, an SCI caused significantly altered levels of eight of these analytes (Fig. 24). Among these, five proinflammatory cytokines/chemokines including IL-la, IL-6, IFN-y, TNF-a, and CCL11 (also known as Eotaxin) were significantly reduced in injured spinal cord tissues from CDldKO as compared to WT mice (Fig. 24); this mainly occurred in the early acute phase of SCI (day 1 post-SCI, Fig. 24). These results are in accordance with the idea that CD Id signaling affects the inflammatory M/M<[) response in the early phase of a SCI. Thus, the reduced levels of inflammatory cytokines/chemokines observed in CDldKO versus WT mouse injured spinal cords are due to the CD Id-deficient environment limiting the development of inflammatory M/M^ in the spinal cord during the early phase of a SCI. Strikingly and of further important note, injured spinal cord tissue from CDldKO mice had higher levels of IL-4, IL-7 and VEGF than in WT mice (Fig. 24). Moreover, the elevated levels of these analytes were maintained from the acute to subacute phase (day 1 - day 3) following a SCI (Fig. 24), in which tissue-repairing M/M$ started to become the dominant subpopulation of M/M<J> overall (Figs. 21-23). IL-4 is a well-known antiinflammatory cytokine and also orchestrates the differentiation of inflammatory M/M$ into tissuerepairing M/M(|). IL-7 directly promotes neuronal survival and neuronal progenitor cell differentiation. VEGF is one of the most important proangiogenic mediators that promote cellular reactions throughout all phases of the wound healing process. Thus, elevated IL-4, IL-7, and VEGF levels in CDldKO spinal cord tissues confer anti-inflammatory and tissue-repairing benefits.
Experiment 5. Spinal cord neuropathology post-injury is not via the conventional
CDld/iNKT cell axis. [00196] CDld not only presents lipid antigens to NKT cells but can also exert intrinsic signaling for regulating immune and inflammatory responses. As it was found that the frequency of NKT cells was extremely low or negligible in spinal cord tissues from WT mice, whether subjected to a SCI or not, it was hypothesized that CDld might contribute to the observed spinal cord neuropathology post-injury in a manner independent of the conventional CDld/NKT cell axis. To test this hypothesis, the effects on SCI outcomes of a-galactosylceramide (a-GalCer) were studied. (a-GalCer is a glycolipid that when presented by CDld strongly activates Type I NKT cells in vivo.) WT mice received a-GalCer or vehicle (PBS) via i.p. injection at 10 minutes after injury, every day of the first week and twice a week from the 2nd week to 6th week post-SCI or sham injury (Fig. 25). The mice were then subjected to the motor and behavioral tests described above. No significant differences in the BMS, Grid-Walking, and Rotarod tests were found between a-GalCer and PBS treatment groups at any of timepoints tested (Figs. 26, 27A-27B). Thus, a-GalCer-triggered activation of Type I NKT cells in vivo does not exhibit a significant impact on the motor and behavioral deficits that were observed in WT mice post-SCI.
[00197] To further clarify the role of the CDld/NKT cell axis in the pathogenesis of SCI, we also studied SCI outcomes in C57BL/6 Traj 18 knockout (Traj 18KO) mice that selectively lack Type I NKT cells due to a genetic deletion in the Traj 18 gene that encodes the J region of their invariant NKT cell receptor a chain. WT and Traj 18KO mice were subjected to a T10 contusive SCI followed by motor and behavioral tests as described above (Fig. 28). Additionally, a tcMMEP test was conducted on the right and left hind limbs of WT and Traj 18KO mice before and after a SCI. No significant differences in the BMS, Grid-Walking, and tcMMEP assessments were found between the groups of WT or Traj l8KO mice at any time point post-SCI (Figs. 29, 30A-30C), although Traj l8KO mice had a shorter latency than WT mice on the Rotarod 5 weeks post-SCI (Figs. 29, 30A-30C). Thus, almost all motor and behavioral measurements are comparable between WT and Traj 18KO mice post-SCI, suggesting that the conventional CD 1 d/iNKT cell axis is unlikely to be involved in the CD Id-mediated spinal cord neuropathology observed following a SCI.
Experiment 6. Administration of an anti-mouse CDld mAb ameliorates CDld-mediated spinal cord neuropathology following a spinal cord injury. [00198] Lipids are an essential structural and functional components of all cell types and tissues in the human body. Particularly, the CNS has a rich lipid composition with about 75% of all mammalian lipid species that are exclusively present in neural tissues, demonstrating the unique requirements of lipids for neural cell functions. In SCI, damaged neural cells and tissues release lipids such as glycolipids that represent a dominant class of lipids in the myelin bilayer of neural cells. Released glycolipids such as CD Id ligands may directly interact with CDld on the surface of CDld+ spinal cord cells (microglia/M$, ODCs, and ECs) to trigger CDld intrinsic signaling in a manner independent of the canonical CDld/NKT cell axis. To clarify whether blockade of CDld- lipid interaction could confer a neuroprotective effect, injected was an anti-mouse CDld mAb [1H6, IgG2b; Roberts et al., Recycling CDldl molecules present endogenous antigens processed in an endocytic compartment to NKT cells. Journal of immunology 168, 5409-5414 (2002).] or isotype (IgG2b) control i.p. to WT mice before and after a SCI (Fig. 31). These mice were subjected to the BMS, Grid-Walking, and Rotarod tests to evaluate the effects of the anti-mouse CDld mAb on motor and behavioral outcomes post-SCI (Figs. 32, 33A-33B). Treatment with the anti-CDld mAb significantly increased BMS scores (Fig. 32), decreased the hind limb foot drop error rate (Fig. 33A), and increased latency on the Rotorod at 6 weeks post-injury (Fig. 33B). Injured spinal cord tissues were collected on day 45 post-SCI for IHC analysis of lesion area size and the amount of SWM (Figs. 34, 35A-35B). Treatment with the anti-CDld mAb significantly reduced the lesion area size and resulted in a greater amount of SWM compared to injured mice receiving the isotype control mAb (Figs. 34, 35A-35B). These results demonstrate that treatment with this anti-CDld mAb has comparable neuroprotective effects as those observed in CDldKO mice, suggesting that a CDld blockade can ameliorate CD Id-mediated spinal cord neuropathology and improve outcomes following a SCI.
Example 2. Generation of Recombinant Human anti-Cdld Monoclonal Antibodies For Treatment of Neurotraumatic Injury.
[00199] In Example 2, monoclonal antibodies are produced, which may be used to specifically bind and thereby inhibit CDld following the occurrence of a neurotraumatic injury such as a SCI. Inhibition of CDld will result in inhibition of the CD Id-mediated inflammation cascade, thereby improving clinical outcomes following incidence of neurotrauma. [00200] The process of generating human monoclonal antibodies for blocking or neutralizing human CDld typically involves several steps, including generation of CDld protein or peptides, immunization, hybridoma production, screening and selection of high-affinity clones, and antibody production and characterization.
[00201] Human CD Id protein or peptides.
[00202] Soluble human CD Id protein or peptides are commercially available. For example, available for purchase is soluble recombinant human CD Id protein from the ProsPec (Quincy, MA; Cat. #: Pro-2491). CDld, produced in Sf9 insect cells, is a single, glycosylated polypeptide chain comprising 290 amino acids (20-301 aa) and having a molecular mass of 32.9kDa (Molecular size on SDS-PAGE will appear at approximately 40-57kDa). CDld is expressed with an 8 amino acid His tag at the C-Terminus and may be purified by proprietary chromatographic techniques. The amino acid sequence of human CDld (SEQ ID No.: 1) is as follows:
EVPQRLFPLR CLQISS FANS SWTRTDGLAW LGELQTHSWS NDSDTVRSLK PWSQGTFSDQ QWETLQHI FR VYRSSFTRDV KEFAKMLRLS YPLELQVSAG CEVHPGNASN NFFHVAFQGK DILS FQGTSW EPTQEAPLWV NLAIQVLNQD KWTRETVQWL LNGTCPQFVS GLLESGKSEL KKQVKPKAWL SRGPSPGPGR
LLLVCHVSGF YPKPVWVKWM RGEQEQQGTQ PGDILPNADE TWYLRATLDV VAGEAAGLSC RVKHSSLEGQ DIVLYWGGSY TSLEHHHHHH
[00203] Immunization.
[00204] Mice are immunized with the human CDld protein or peptide as an antigen, together with an aluminum-containing adjuvant to elicit an immune response in the mice.
[00205] Isolation of B cells.
[00206] B cells are isolated from spleen of the immunized mice using techniques such as density gradient centrifugation, magnetic bead separation, or fluorescence-activated cell sorting (FACS).
[00207] Stimulation and cloning of B cells. [00208] Once isolated, the B cells are diluted at 1 cell per 200 pL of culture medium (Roswell Park Memorial Institute (RPMI) 1640 Medium, supplemented with fetal bovine serum and other growth factors) and then added to 96- well microplates with 200 pL per well (1 B cell per well). The culture medium includes a combination of cytokines and mitogens, such as interleukin-2 (IL -2), interleukin-4 (IL-4), interleukin- 10 (IL-10), and CD40 ligand.
[00209] Co-culture with feeder cells.
[00210] B cells may be co-cultured with specialized feeder cells, such as the murine 3T3 fibroblast cell line, which provide growth factors and cytokines to support expansion of B cells.
[00211] Screening antibody-producing B cells.
[00212] Supernatant fluids from each well are harvested for testing for anti-CDld antibody titre and neutralizing activity.
[00213] Isolation of antibody genes.
[00214] Antibody-coding RNA are extracted from the B cell clones that produce high titres of blocking and/or neutralizing anti-CDld antibodies. The genes encoding such monoclonal antibodies are amplified, isolated, sequenced, and cloned using standard techniques.
[00215] Humanization of antibody genes.
[00216] The variable regions of the antibody genes are modified to create humanized versions. This may be done by replacing he mouse-derived constant regions with human constant regions, while retaining the variable regions.
[00217] Cloning of the humanized antibody genes.
[00218] The humanized antibody are cloned into expression vectors that can be used to produced large quantities of the antibody.
[00219] Expression of the humanized antibody. [00220] The cloned expression vector(s) are introduced into host cells such as Chinese hamster ovary (CHO) cells, which are then grown in culture to produce large quantities of the humanized antibody.
[00221] Antibody purification and testing.
[00222] The humanized monoclonal antibody is purified from the culture medium and tested for its specificity and effectiveness in targeting CD Id.
Example 3. Treating Neurotraumatic Injury Using Cdld Inhibitor.
[00223] The safety and efficacy of purified anti-CDld antibody and/or antibody fragment in treatment of neurotraumatic are tested in a series of preclinical animal trials followed by human clinical trials.
[00224] Human clinical trials are double-blinded and randomized. Trial subjects may be qualified adults aged 18 years to 64 years assigned within 24 hours of their traumatic injury. (Compare with study NCT01322048.)
[00225] Following a qualifying neurotraumatic injury, e.g., a SCI, patient are infused with a dose of anti-CDld antibody or fragment, or placebo. Primary outcome measure for SCI incidence are improved neuromotor function from injury baseline. Secondary outcome measure are reduced cerebrospinal fluid inflammatory analyte levels, including IL- la, IL-6, IFN-y, TNF-a, and CCL11.