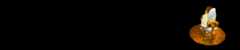
Tests of Big Bang: The CMB
TheBig Bang theory predicts that the early universe was avery hot place and that as it expands, the gas within it cools. Thus the universe shouldbe filled with radiation that is literally the remnant heat left over from the Big Bang,called the cosmic microwave background", or CMB.
Discovery of the Cosmic Microwave Background
The existence of the CMB radiation was first predicted by Ralph Alpherin 1948 in connection with his research onBig Bang Nucleosynthesis undertaken together with Robert Herman and George Gamow. It was first observed inadvertently in 1965 byArno Penzias and Robert Wilson at the Bell Telephone Laboratories in Murray Hill, NewJersey. The radiation was acting as a source of excess noise in a radio receiver they werebuilding. Coincidentally, researchers at nearby Princeton University, led by Robert Dickeand including Dave Wilkinson of the WMAP science team, were devising an experiment to findthe CMB. When they heard about the Bell Labs result they immediately realized that the CMBhad been found. The result was a pair of papers in the Astrophysical Journal (vol. 142 of 1965): one by Penzias andWilson detailing the observations, and one by Dicke, Peebles, Roll, and Wilkinson givingthe cosmological interpretation. Penzias and Wilson shared the 1978 Nobel prize in physicsfor their discovery.
Today, the CMB radiation is very cold, only 2.725° aboveabsolute zero, thus this radiation shines primarilyin the microwave portion of theelectromagneticspectrum, and is invisible to the naked eye. However, it fills the universe and can bedetected everywhere we look. In fact, if we could see microwaves, the entire sky wouldglow with a brightness that was astonishingly uniform in every direction. The picture atleft shows a false color depiction of the temperature (brightness) of the CMB over thefull sky (projected onto an oval, similar to a map of the Earth). The temperature isuniform to better than one part in a thousand! This uniformity is one compelling reason tointerpret the radiation as remnant heat from the Big Bang; it would be very difficult toimagine a local source of radiation that was this uniform. In fact, many scientists havetried to devise alternative explanations for the source of this radiation, but none havesucceeded.
Why study the Cosmic Microwave Background?
Since light travels at a finite speed, astronomers observing distant objects arelooking into the past. Most of the stars that are visible to the naked eye in the nightsky are 10 to 100 light years away. Thus, we see them as they were 10 to 100 years ago. Weobserve Andromeda, the nearest big galaxy, as it was about 2.5 million years ago.Astronomers observing distant galaxies with the Hubble Space Telescope can see them asthey were only a few billion years after the Big Bang.
The CMB radiation was emitted 13.7 billion years ago, only a few hundred thousand years after the Big Bang,long before stars or galaxies ever existed. Thus, by studying the detailed physicalproperties of the radiation, we can learn about conditions in the universe on very largescales at very early times, since the radiation we see today has traveled over such a large distance.
The Origin of the Cosmic Microwave Background
One of the profound observations of the 20th century is that the universe isexpanding. This expansion implies the universe wassmaller, denser and hotter in the distant past. When the visible universe was half itspresent size, the density of matter was eight times higher and the cosmic microwavebackground was twice as hot. When the visible universe was one hundredth of its presentsize, the cosmic microwave background was a hundred times hotter (273 degrees aboveabsolute zero or 32 degrees Fahrenheit, the temperature at which water freezes to form iceon the Earth's surface). In addition to this cosmic microwave background radiation, theearly universe was filled with hot hydrogen gas with a density of about 1000 atoms percubic centimeter. When the visible universe was only one hundred millionth its presentsize, its temperature was 273 million degrees above absolute zero and the density ofmatter was comparable to the density of air at the Earth's surface. At these hightemperatures, the hydrogen was completely ionized into free protons and electrons.
Since the universe was so very hot through most of its early history, there were noatoms in the early universe, only free electrons and nuclei. (Nuclei are made of neutronsand protons). The cosmic microwave background photons easily scatter off of electrons.Thus, photons wandered through the early universe, just as optical light wanders through adense fog. This process of multiple scattering produces what is called athermal or blackbody spectrum of photons. According to the BigBang theory, the frequency spectrum of the CMB should have this blackbody form. This wasindeed measured with tremendous accuracy by the FIRAS experiment on NASA's COBEsatellite.
This figure shows the prediction of the Big Bang theory for the energy spectrum of thecosmic microwave background radiation compared to the observed energy spectrum. Specifically a measurement was made of the surface brightness per unit frequency interval (𝛪𝜈), not 𝛪𝜆 - which is a per unit wavelength interval. The FIRAS experiment measured the spectrum at 34 equally spaced points along the blackbody curve.The error bars on the data points are so small that they can not be seen under thepredicted curve in the figure! There is no alternative theory yet proposed that predictsthis energy spectrum. The accurate measurement of its shape was another important test ofthe Big Bang theory.
Surface of Last Scattering
Eventually, the universe cooled sufficiently that protons and electrons could combineto form neutral hydrogen. This occured roughly 400,000 years after the BigBang when the universe was about one eleven hundredth its present size. Cosmic microwavebackground photons interact very weakly with neutral hydrogen, allowing them to travel in a straight lines.
The behavior of CMB photons moving through the early universe is analogous to thepropagation of optical light through the Earth's atmosphere. Water droplets in a cloud arevery effective at scattering light, while optical light moves freely through clear air.Thus, on a cloudy day, we can look through the air out towards the clouds, but can not seethrough the opaque clouds. Cosmologists studying the cosmic microwave background radiationcan look through much of the universe back to when it was opaque: a view back to 380,000years after the Big Bang. This wall of light is called the surface of lastscattering since it was the last time most of the CMB photons directly scattered off ofmatter. When we make maps of the temperature of the CMB, we are mapping this surface oflast scattering.
As shown above, one of the most striking features about the cosmic microwave backgroundis its uniformity. Only with very sensitive instruments, such asCOBE andWMAP, can cosmologists detectfluctuations in the cosmic microwave background temperature.By studying these fluctuations, cosmologists can learn aboutthe origin of galaxies and large scale structures ofgalaxies and they can measure the basicparameters of the Big Bang theory.