Hippocampal sharp wave bursts coincide with neocortical "up-state" transitions
- PMID:15576887
- PMCID: PMC534698
- DOI: 10.1101/lm.73504
Hippocampal sharp wave bursts coincide with neocortical "up-state" transitions
Abstract
The sleeping neocortex shows nested oscillatory activity in different frequency ranges, characterized by fluctuations between "up-states" and "down-states." High-density neuronal ensemble recordings in rats now reveal the interaction between synchronized activity in the hippocampus and neocortex: Electroencephalographic sharp waves in the hippocampus were more probable during down-states than during up-states, and tended to coincide with transitions from down-states to up-states. The form of cortical activity fluctuations and their interactions with sharp waves depend on sleep depth: In deeper sleep stages, characterized by strong neocortical oscillation in the delta range or slower (approximately 0.8-4 Hz), sharp-wave-triggered peri-event time histograms (PETH) are consistent with a longer duration for down-states than for up-states. In lighter sleep, the sharp-wave-triggered PETH suggested longer up-states than down-states. These results highlight the interplay in the hippocampal/neocortical loop: Decreased neocortical input during down-states may be a factor in generation of sharp waves. In turn, sharp waves may facilitate down-to-up transitions. This interplay may reflect joint memory trace reactivation in the hippocampus and in the neocortex, possibly contributing to consolidation of long-term memory: Off-line reactivation of recent neural activity patterns in the hippocampus occurs during 50-100-msec electroencephalographic sharp waves, corresponding to pyramidal-cell population bursts. The neocortical up-states starting in correspondence with sharp waves may be influenced by the reactivated information carried by the hippocampal sharp wave.
Figures
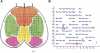
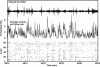
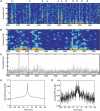
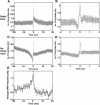
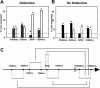
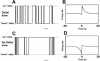
Similar articles
- Slow-wave sleep and the consolidation of long-term memory.Born J.Born J.World J Biol Psychiatry. 2010 Jun;11 Suppl 1:16-21. doi: 10.3109/15622971003637637.World J Biol Psychiatry. 2010.PMID:20509828Review.
- Hippocampal sharp wave-ripples linked to slow oscillations in rat slow-wave sleep.Mölle M, Yeshenko O, Marshall L, Sara SJ, Born J.Mölle M, et al.J Neurophysiol. 2006 Jul;96(1):62-70. doi: 10.1152/jn.00014.2006. Epub 2006 Apr 12.J Neurophysiol. 2006.PMID:16611848
- Integration and segregation of activity in entorhinal-hippocampal subregions by neocortical slow oscillations.Isomura Y, Sirota A, Ozen S, Montgomery S, Mizuseki K, Henze DA, Buzsáki G.Isomura Y, et al.Neuron. 2006 Dec 7;52(5):871-82. doi: 10.1016/j.neuron.2006.10.023.Neuron. 2006.PMID:17145507
- NREM sleep in the rodent neocortex and hippocampus reflects excitable dynamics.Levenstein D, Buzsáki G, Rinzel J.Levenstein D, et al.Nat Commun. 2019 Jun 6;10(1):2478. doi: 10.1038/s41467-019-10327-5.Nat Commun. 2019.PMID:31171779Free PMC article.
- Hippocampal-cortical interactions and the dynamics of memory trace reactivation.Schwindel CD, McNaughton BL.Schwindel CD, et al.Prog Brain Res. 2011;193:163-77. doi: 10.1016/B978-0-444-53839-0.00011-9.Prog Brain Res. 2011.PMID:21854962Review.
Cited by
- Cell Assemblies in the Cortico-Hippocampal-Reuniens Network during Slow Oscillations.Angulo-Garcia D, Ferraris M, Ghestem A, Nallet-Khosrofian L, Bernard C, Quilichini PP.Angulo-Garcia D, et al.J Neurosci. 2020 Oct 21;40(43):8343-8354. doi: 10.1523/JNEUROSCI.0571-20.2020. Epub 2020 Sep 29.J Neurosci. 2020.PMID:32994338Free PMC article.
- Abnormal UP/DOWN Membrane Potential Dynamics Coupled with the Neocortical Slow Oscillation in Dentate Granule Cells during the Latent Phase of Temporal Lobe Epilepsy.Ouedraogo DW, Lenck-Santini PP, Marti G, Robbe D, Crépel V, Epsztein J.Ouedraogo DW, et al.eNeuro. 2016 May 31;3(3):ENEURO.0017-16.2016. doi: 10.1523/ENEURO.0017-16.2016. eCollection 2016 May-Jun.eNeuro. 2016.PMID:27257629Free PMC article.
- Priming of hippocampal population bursts by individual perisomatic-targeting interneurons.Ellender TJ, Nissen W, Colgin LL, Mann EO, Paulsen O.Ellender TJ, et al.J Neurosci. 2010 Apr 28;30(17):5979-91. doi: 10.1523/JNEUROSCI.3962-09.2010.J Neurosci. 2010.PMID:20427657Free PMC article.
- Occurrence of Hippocampal Ripples is Associated with Activity Suppression in the Mediodorsal Thalamic Nucleus.Yang M, Logothetis NK, Eschenko O.Yang M, et al.J Neurosci. 2019 Jan 16;39(3):434-444. doi: 10.1523/JNEUROSCI.2107-18.2018. Epub 2018 Nov 20.J Neurosci. 2019.PMID:30459228Free PMC article.
- Sleep and synaptic plasticity in the developing and adult brain.Frank MG.Frank MG.Curr Top Behav Neurosci. 2015;25:123-49. doi: 10.1007/7854_2014_305.Curr Top Behav Neurosci. 2015.PMID:24671703Free PMC article.Review.
References
- Achermann, P. and Borbely, A.A. 1997. Low-frequency (<1 Hz) oscillations in the human sleep electroencephalogram. Neuroscience 81: 213-222. - PubMed
- Ball, G.J., Gloor, P., and Schaul, N. 1977. The cortical electromicrophysiology of pathological delta waves in the electroencephalogram of cats. Electroencephalogr. Clin. Neurophysiol. 43: 346-361. - PubMed
- Battaglia, F.P, Sutherland, G.R., and McNaughton, B.L. 2001. Widespread modulation of neocortical cell activity during hippocampal sharp waves. Soc. Neurosci. Abs. 27: 643.16.
- Buzsaki, G. 1989. Two-stage model of memory trace formation: A role for “noisy” brain states. Neuroscience 31: 551-570. - PubMed
- Buzsaki, G., Horvath, Z., Urioste, R., Hetke, J., and Wise, K. 1992. High-frequency network oscillation in the hippocampus. Science 256: 1025-1027. - PubMed
Publication types
MeSH terms
Grants and funding
LinkOut - more resources
Full Text Sources
Other Literature Sources